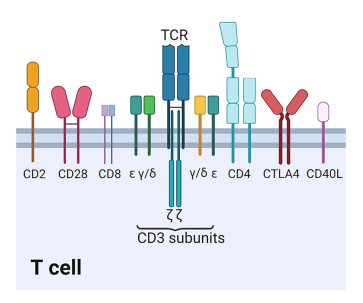
Citation: | Hong-fei Tian, Jing Xing, Xiao-qian Tang, Heng Chi, Xiu-zhen Sheng, Wen-bin Zhan. 2022: Cluster of differentiation antigens: essential roles in the identification of teleost fish T lymphocytes. Marine Life Science & Technology, 4(3): 303-316. DOI: 10.1007/s42995-022-00136-z |
Leukocytes express distinct assortments of molecules on their cell surfaces. These surface molecules play critical roles in signaling and many reflect different stages of leukocytes lineage-specific differentiation (Cruse et al. 2004). Antibodies that react with cell surface antigens are known to be one of the most essential tools for identifying leukocyte subpopulations. Therefore, in the early years, large numbers of monoclonal antibodies (mAbs) that react to these cell surface molecules were developed by immunologists, each with different associated nomenclatures (Zola et al. 2005). In the absence of a unified nomenclatural system for mAbs, it was very difficult to tell if more than one antibody was specific for the same molecule (Zola et al. 2003). In the 1980s, the establishment of the human leukocyte differentiation antigens (HLDA) workshop brought order to the chaos (Bernard and Boumsell 1984; Boumsell 1996). A standard nomenclature for several mAbs that react with a specific antigen has been implemented, which provides consistency and uniformity when referring to the same molecules (Engel et al. 2015). Clusters of antigens on the surface of leukocytes can be clearly designated by their reactions with mAbs. This designation of antigens is called clusters of differentiation (CDs), and this common nomenclature has been applied not only to human, but also to other vertebrates, including teleost fish. To date, more than 400 human proteins have been designated as CD markers, and the HLDA workshops continue to be held on a 4-year cycle with the main purpose of updating newly characterized molecules (Zola et al. 2005).
T lymphocytes, as an important population of leukocytes, play essential roles in the adaptive immune system. Many of the CD antigens expressed on T lymphocytes are involved in signal transduction and activation, and several CD antigens can be used as cell surface markers of T lymphocyte classification, including CD3, CD4, CD8, CD28 and CTLA4 (CD152) (Nakanishi et al. 2015). For example, CD3 and TCR molecules coexist on the surface of T cells, forming the basic structure of T cell antigen-specific recognition and cell activation signal transmission (Ashwell and Klausner 1990; Klausner et al. 1990). T lymphocytes can be divided into T helper (Th) and T cytotoxic (Tc) cells, which are distinguished by the expression of CD4 and CD8 glycoproteins, respectively (Kato et al. 2013). The counter-receptors of CD28 and CTLA4 are important T cell costimulatory receptors and involved in the activation or inactivation of T cells (Chen and Flies 2013). Teleost fish, the oldest vertebrate group, exhibit all the major features of the mammalian immune system and have both innate and adaptive immunity. T lymphocytes and their subsets have been found and identified in fish. According to the definition and nomenclature of CD antigens in mammals, many of CD molecules expressed on T lymphocytes also have been cloned and identified in multiple fish species (Castro et al. 2011). Together with the development of mAbs that recognize surface molecules on T lymphocytes, the phenotypes and properties of T cells have become important issues for fish immunologists. Two subpopulations, CD4+ and CD8+ T lymphocytes (functionally identified as Th and Tc cells in mammals) have also been found and characterized in fish (Nakanishi et al. 2015). Furthermore, CD2, CD28, CTLA4 and other cluster differentiation antigens have also been characterized in teleost fish (Bernard et al. 2007; Cho et al. 2017; Hu et al. 2012; Jeswin et al. 2017; Shao et al. 2018). However, the precise roles of fish CD antigens in the classification, signal transduction and activation of T cells are still unclear.
In this review, we summarize recent progress in the identification of CD molecules on T cells in teleost fish, with emphasis on the functions of CD markers in the classification of fish T lymphocyte subsets. The main aims are to deepen our understanding of the precise role of fish T lymphocyte subpopulations in adaptive immunity, and to facilitate the health management and development of vaccines for fish.
CD3 molecules coexist in the form of TCR-CD3 complex on T lymphocytes, and this complex is made up of an αβ or γδ heterodimer of TCR and the subunits (γ, δ, ɛ and ζ chains) of CD3 (Kim and Park 2005; Park et al. 2001). The TCR-CD3 complex plays essential roles in specific antigen recognition, cell activation and signal transmission in T cells (Jung et al. 2017; Kuhns et al. 2006). Structurally, the γ, δ and ɛ chains of CD3 are members of the immunoglobulin (Ig) superfamily and consist of an extracellular Ig-like domain, a transmembrane helix and a cytoplasmic tail, whereas the CD3ζ chain has a short extracellular peptide, a transmembrane part and a long cytoplasmic tail (Liu et al. 2008). All the CD3 subunits contain immunoreceptor tyrosine-based activation motifs (ITAMs) in the intracellular domains that connect with tyrosine kinases during the signal transduction (Kuhns et al. 2006; Randelli et al. 2011). Similar to mammals, four T cell receptor genes, which encode the TCR α-, β-, γ- and δ-chains, and three CD3 chains (CD3γ/δ, -ɛ and -ζ), have been cloned in teleost fish (Fig. 1), thereby displaying the conservation of functional characteristics (Langenau and Zon 2005; Maisey et al. 2011). The CD3γ/δ gene that corresponds to the forerunner of mammalian CD3γ and CD3δ genes has been reported in multiple fish species, as well as from birds and amphibians, suggesting a common ancestor of mammalian CD3γ and CD3δ (Araki et al. 2005; Kim and Park 2005; Liu et al. 2008; Park et al. 2001; Randelli et al. 2011; Shang et al. 2008).
In mammals, the TCR-CD3 complex has been the focus of intense research covering aspect of biosynthesis, assembly, structure and signaling, which has given insights into the roles of T cells in immunity (Göbel and Dangy 2000). Although the CD3 subunits have been reported in multiple fish species, the research was focused on gene cloning rather than structure or signaling (Kim and Park 2005). However, CD3 molecules are essential cell surface markers of T lymphocytes, and the antibodies against these molecules are powerful tools to identify T lymphocytes and study their immune properties in fish. In earlier studies, anti-human CD3 antibodies have been used to react with CD3 molecules in different mammalian, avian and even fish species, when the sequence information of non-mammalian CD3 proteins were not available (Bertram et al. 1996; Cook et al. 2001; Keresztes et al. 1996; Wilkinson et al. 1995). ZAP70, a tyrosine kinase protein (70 kDa), expressed in T cells and crucial for their selective activation, has also been used to label fixed T cells in fish by using anti-human ZAP70 mAbs (Yoon et al. 2015). With the need of research and development of sequencing, mAbs that specifically recognized fish T cells have been developed (Fig. 2). The mAbs of DLT15 were developed that recognize thymocytes and T lymphocytes in peripheral tissues of sea bass (Dicentrarchus labrax), and the mAbs of WCL38 were produced to recognize intestinal T cells in the common carp (Cyprinus carpio) (Rombout et al. 1998; Scapigliati et al. 2000). The antibodies or RNA probes against TCR molecules were also used to label T lymphocytes in fish (Picchietti et al. 2008; Romano et al. 2011; Timmusk et al. 2003). In addition, the CD3 subunit complex is specifically expressed on the surface of T cells, and the generation of antisera or mAbs against fish CD3 chains are powerful tools for identifying T cells (Table 1). In salmon, plenty of CD3ɛ+ T cells in the thymus, intestine and gill were identified using the antisera against a synthetic peptide of the CD3ɛ chain (Koppang et al. 2010). Subsequent morphological analysis revealed that T cells aggregated in the thymus, spleen, and even in the interbranchial lymphoid tissue of salmon. The results suggest that the interbranchial lymphoid tissue in fish is an important location of T cell aggregation and for facilitating the encounter of antigens (Koppang et al. 2010).
Marker molecules | Fish species | Type of antibody | Application | References |
CD3ɛ | Salmon | pAb | WB, FCM, IHC, IFA | Koppang et al. (2010) |
Trout | mAb | Co-IP, FCM, IFA | Boardman et al. (2012) | |
Salmon | pAb | WB, FCM, IFA | Maisey et al. (2016) | |
Flounder | pAb | Co-IP, FCM, IFA, IHC | Tang et al. (2017) | |
Flounder | mAb | WB, FCM, IFA, FACS | Jung et al. (2017) | |
CD4-1 | Spotted green pufferfish | pAb | WB | Wen et al. (2011) |
Ginbuna crucian carp | mAb | WB, FCM, IFA, FACS | Toda et al. (2011) | |
Japanese pufferfish | pAb | WB, FCM, IFA, FACS | Kono and Korenaga (2013) | |
Zebrafish | pAb | WB, IFA, IHC, FCM, FACS | Yoon et al. (2015) | |
Trout | mAb | WB, FCM, IFA, FACS | Takizawa et al. (2016) | |
Trout | pAb | WB, FCM, IFA, FACS | Maisey et al. (2016) | |
Flounder | pAb mAb |
WB, FCM, IFA, IHC, FACS WB, FCM, IFA, FACS |
Xing et al.(2017a, b) Xing et al. (2020) |
|
Ginbuna crucian carp | pAb | IFA | Kato et al. (2019) | |
Flounder | mAb | WB, FCM, IFA, FACS | Jung et al. (2020a) | |
CD4-2 | Spotted green pufferfish | pAb | WB, FCM, IHC, FACS | Wen et al. (2011) |
Trout | mAb | WB, FCM, IFA, FACS | Takizawa et al. (2016) | |
Flounder | pAb mAb |
WB, FCM, IFA, IHC, FACS WB, FCM, IFA, FACS |
Xing et al.(2017a, b) Xing et al. (2020) |
|
Flounder | mAb | WB, FCM, IFA, FACS | Jung et al. (2020b) | |
CD8α | Torafugu | pAb | WB, Co-IP, FCM, FACS | Araki et al. (2008) |
Ginbuna crucian carp | mAb | WB, FCM, IFA, FACS | Toda et al. (2009) | |
Salmon | mAb | IHC | Hetland et al. (2010) | |
Orange-spotted grouper | pAb | WB, FCM, IFA | Chang et al. (2011) | |
Trout | mAb | WB, Co-IP, FCM, IFA, IHC, FACS | Takizawa et al. (2011) | |
Flounder | mAb | WB, FCM, IFA, FACS | Jung et al. (2021) | |
CD8β | Flounder | pAb | WB, FCM, IFA, IHC, FACS | Xing et al.(2017a, b) |
Flounder | mAb | WB, FCM, IFA, FACS | Jung et al. (2021) | |
Co-IP Co-immunoprecipitation, WB Western blotting, FCM Flow cytometry, IFA Immunofluorescence assay, IHC Immunohistochemistry, FACS Fluorescence activated cell sorting |
Boardman et al. (2012) prepared a monoclonal antibody against the peptide in the cytoplasmic tail region of CD3ɛ protein in trout (Oncorhynchus mykiss) and found that CD3ɛ+ cells were most abundant in the thymus and skin but did not co-locate with IgM+ cells (Boardman et al. 2012). The antibodies against the intracellular sequences of CD3ɛ chain have been used to identify salmon and trout CD3+ T cells in fixed or permeabilized cells (Boardman et al. 2012; Koppang et al. 2010). Furthermore, the extracellular peptides of CD3 molecules were also selected for antibody production in Atlantic salmon and flounder (Paralichthys olivaceus), respectively (Jung et al. 2017; Maisey et al. 2016; Tang et al. 2017). In a previously study, flounder CD3+ T cells were observed in tissues and no cross-reaction with mIgM+ B cells were found. Furthermore, the percentages of CD3+ T lymphocytes sharply increased after immunization with inactivated Edwardsiella tarda (Tang et al. 2017). Jung et al. (2017) also produced a monoclonal antibody against the CD3ɛ chain in flounder, and the isolated CD3+ T cells detected the expression of related genes on T cells, such as TCR, CD4 and CD8 (Jung et al. 2017). CD3+ T cells have also been targeted directly with in situ hybridization in fish, and the results showed that fugu CD3 positive cells were detected in kidney, spleen and thymus (Araki et al. 2005).
According to the composition of TCR chains, CD3+ T lymphocytes can be subdivided into αβ and γδ T cells (Wan et al. 2017). Conventional αβ T cells are the more plentiful T cell type (90-95%), circulating in lymphoid organs and blood, and can be classified as CD4+ and CD8+ T cells depending on the surface glycoproteins (Hayday 2000; Prinz et al. 2013; Silva-Santos et al. 2015). CD4+ and CD8+ T cells in teleost fish will be the main focus of attention in the following sections. Among this small group of cells, which represent only 5-10% of T cells, mammalian γδ T cells are the most primitive immune cells and are mainly found in epithelial and mucosal tissues. γδ T cells have been identified and functionally characterized only in zebrafish (Wan et al. 2017). Therefore, further studies on the immune properties of fish γδ T cells and their functional differences with αβ T cells are needed.
CD4, a co-receptor of T cells belonging to the immunoglobulin superfamily (IgSF), has been cloned in many fish species (Ashfaq et al. 2020; Buonocore et al. 2008; Edholm et al. 2007; Kato et al. 2013; Laing et al. 2006; Maisey et al. 2011; Mao et al. 2017; Patel et al. 2009; Sun et al. 2007; Tran et al. 2020). In mammals, a single CD4 gene with four extracellular Ig-like domains (D1-D4) was characterized, whereas two CD4 molecules (CD4-1 and CD4-2) were found in teleost fish (Fig. 3). CD4-1 contains four extracellular Ig-like domains (D1-D4), similar to single CD4 gene in mammals, and a distinct CD4-2 gene that contains two or three Ig-like domains (D1-D2 or D1-D3) (Kato et al. 2013; Takizawa et al. 2016). CD4-1 is present in all teleost fish species studied to date. CD4-like molecules with two Ig-like domains have been identified in rainbow trout, Atlantic salmon, fugu, Atlantic halibut and flounder, whereas CD4-like molecule with three Ig-like domains has only been reported in catfish (Ashfaq et al. 2020; Castro et al. 2011). CD4 molecules show low amino acid identity between fish and higher vertebrates; however, the characteristics of gene structure, splicing patterns, binding motifs and key residues are conserved, and the gene features of CD4 in teleosts have been well documented (reviewed by Ashfaq et al. 2019; Castro et al. 2011). Based on the organization of Ig-like domains in CD4-1 (V-C-V-C) and CD4-2 (V-C or V-C-V), it has been hypothesized that the four-domain CD4 molecule could have arisen by the duplication of an ancestral two-domain (V-C) receptor (Baixeras et al. 1992; Laing and Hansen 2011; Laing et al. 2006; Triebel et al. 1990; Williams et al. 1989). Furthermore, a lamprey CD4-like gene with only two Ig-like domains (V-C) has been reported and thought to be the primordial two Ig-like domain CD4 molecule in vertebrates (Pancer et al. 2004).
Except a long extracellular region, CD4 in mammals contains a transmembrane domain and a critical cytoplasmic tail with a conserved motif (CXC) that interacts with the tyrosine kinase Lck (Kim et al. 2003; Moore et al. 2009; Turner et al. 1990). During antigen recognition, the extracellular D1 and D2 region of CD4 binds to the MHC class II complex expressed on antigen-presenting cells (APCs), and then the cytoplasmic tail of CD4 noncovalently connects with the Lck protein tyrosine kinase, initiating T cell activation (Maisey et al. 2016; Merwe and Davis 2002; Salmond et al. 2010). The cytoplasmic motif was also found in both fish CD4-1 and CD4-2 molecules, and is thought to participate in the activation of T lymphocytes after association with Lck (Moore et al. 2009). This phenomenon suggests that both teleost CD4 coreceptors have a similar function to mammalian CD4 molecules and engage in the development and activation of T cells. Interestingly, the genes encoding CD4 were not found in the Atlantic cod (Gadus morhua) and other gadiform species, showing a unique immune system (Malmstrøm et al. 2016; Star et al. 2011). In cobia (Rachycentron canadum), the transmembrane domain and CXC motif are not present in the deduced CD4-2b protein, which suggests a difference in CD4-2b activity (Tran et al. 2020). It is noteworthy that this was the first record of soluble CD4 molecules in fish. In conclusion, although CD4 genes have been cloned in multiple fish species, further studies are needed to determine the details of their expression and function in signaling.
In addition to being a co-receptor in T cells involved in signal transduction, CD4 is also considered as an important surface antigen for identifying Th cells (Wan and Flavell 2009). In fish, recombinant proteins or synthetic peptides were selected and generated as immunogens for the development of monoclonal antibodies or polyclonal antibodies recognizing CD4 molecules (Xing et al. 2017a; Yoon et al. 2015). The function of CD4 in signal transduction has not been elucidated, but there are many studies on the use of CD4 as T cell surface markers to identify CD4+ Th cells (Table 1). CD4+ T cells were identified in spotted green pufferfish (Tetraodon nigroviridis) at the cellular level (Wen et al. 2011). Furthermore, a CD4-2+CD25-like+Foxp3-like+ Treg-like cell population was first identified and functionally characterized in a pufferfish (Wen et al. 2011). Consequently, after the depletion of Treg-like cells, the nonspecific cytotoxic cell (NCC) activity, mixed lymphocyte reaction (MLR) and inflammation in the intestine are all enhanced in pufferfish (Wen et al. 2011). The mAbs (6D1, 9F1 and 2C1) against CD4-1 have been produced in carp (Carassius auratus langsdorfii) to trace CD4+ T cells (Toda et al. 2011). In addition, many functional studies have been carried out using the mAbs in carp (reviewed by Nakanishi et al. 2015), and it was suggested that CD4+ T lymphocytes in carp function as Th cells in mammals (Nayak and Nakanishi 2013; Shibasaki et al. 2010; Somamoto et al. 2014a, b; Yamasaki et al. 2014). In Japanese pufferfish (Takifugu rubripes), high-purity CD4+ cells were isolated using a specific anti-CD4 antibody, and T cell surface markers, not B cell or macrophage marker genes, were expressed on sorted CD4+ T cells (Kono and Korenaga 2013). In addition, the expression of Th1, Th17, and Treg cytokines in Japanese pufferfish CD4+ T cells is up-regulated by stimulation after Lipopolysaccharides (LPS) and Polyinosinic-polycytidylic acid (Poly(I: C)), while Th2 cytokines are down-regulated, indicating that these CD4+ cells have a similar profile of Th-type cytokine secretion like in mammals (Kono and Korenaga 2013). Similarly, CD4-1+ T lymphocytes were identified by using a polyclonal antibody against CD4 molecules in zebrafish (Danio rerio) and the results showed that the expression of master transcription factors and cytokines related to Th1 or Th2-type responses were increased after antigen specific stimulation (Yoon et al. 2015). Takizawa et al. (2016) generated mAbs against trout (Oncorhynchus mykiss) CD4-1 and CD4-2 molecules and characterized three CD4+ leukocytes (CD4-1/CD4-2 double-positive, CD4-2 single-positive T cells and CD4-1 single-positive monocyte/macrophage populations). In the same study, after infection with Yersinia ruckeri, CD4+ T lymphocytes generated equivalent levels of cytokines relevant to Th1, Th17, and regulatory T cells, and CD4+ monocyte/macrophage populations had high phagocytic capacity. CD4-1+ subpopulations of T cells in trout were also reported in another study by using polyclonal antibodies against a peptide from the trout CD4-1 sequence (Maisey et al. 2016). Additionally, a long-term CD4-1+ T lymphocyte line was established and assessed in trout using IL-15 as a growth factor (Maisey et al. 2016). In a previous study, we identified CD4+ T lymphocytes in peripheral blood, spleen and head kidney from flounder, and CD4-1+/CD4-2+ T lymphocytes were identified in the majority populations among three of CD4+ T lymphocyte subsets (Xing et al. 2017a). Flounder CD4+ T cells were also found to respond to specific immunostimulants, up-regulated cytokines, and transcription factors of Th subsets (Xing et al. 2020). Similarly, Jung et al.(2020a, b) investigated the cellular immune response of CD4-1+ and CD4-2+ T cells after infection with viral hemorrhagic septicemia virus (VHSV) and nervous necrosis virus (NNV) in flounder. A polyclonal antibody anti-CD4-1 was also developed in ginbuna crucian carp (Carassius auratus langsdorfii) to analyse the immunity of CD4-1+ cell in the granulomatous inflammation against mycobacterial infections (Kato et al. 2019).
Although CD4+ T lymphocytes have been extensively characterized in several teleost fish, their precise functions have been only superficially studied. In mammals, CD4+ T lymphocytes can differentiate into one of several lineages of Th cell subsets that produce multiple cytokines, which participate in the regulation of inflammation and responses against different pathogens (Zhou et al. 2009; Zhu and Paul 2010; Zhu et al. 2010). In CD4+ T cell subsets, there is increasing evidence that the function of Th cell subsets in teleosts is the same as that in mammals (reviewed by Ashfaq et al. 2019; Castro et al. 2011; Fischer et al. 2013; Nakanishi et al. 2015; Tafalla et al. 2016). In a previous study, we found that after the suppression of T lymphocytes, especially CD4+ T lymphocytes, the immune responses of B lymphocytes were distinctly inhibited, which suggests that CD4+ T lymphocytes regulate the immune response of mIgM+ B cells in flounder (Xing et al. 2017b, 2019b). In other fish species, CD4+ T cells were also involved in a variety of immune functions, such as stimulating macrophages to increase microbicidal activity, B lymphocytes to produce antibodies and enhancing cell-mediated immunity (Nakanishi et al. 2015). In addition, the genes that encode unique transcription factors and hallmark cytokines of helper T cell subsets are represented in most teleost genomes (Wang et al. 2010). There are ample evidences that CD4+ T lymphocytes up-regulate the expression of master transcription factors and cytokines relevant to Th-type responses following antigen specific stimulation in fish (Kono and Korenaga 2013; Takizawa et al. 2016; Tian et al. 2021; Xing et al. 2020; Yoon et al. 2015). These studies support the potential existence of effector T cell subsets in fish (Fig. 4). However, functional studies on CD4+ T lymphocytes are far from sufficient and it is difficult to detect CD4+ T lymphocytes biased toward a specific Th phenotype in fish. In mammals, CD4 is also found on the surface of other leukocytes, for example, human and rat monocytes/macrophages, but not on monocytes/macrophages from mice and birds (Chan et al. 1988; Gordon and Taylor 2005). Similarly, CD4-1+ myeloid cell populations with the highest recorded phagocytic activity and capacity were described in trout (Takizawa et al. 2016), CD4-1 and CD4-2 genes in zebrafish were found not only in lymphocytes but also in monocytes/macrophages and even precursor cells (Yoon et al. 2015). In a previous study, we also found that sorted flounder CD4+ cells express CSF-1R, a surface marker of monocytes/macrophages (Xing et al. 2020). In salmon, it has not been well explained that cd8α, cd8β and IgM genes were expressed in sorted CD4+ cells (Maisey et al. 2016). Furthermore, it has been shown that the CD4+ T cells in carp (Carassius auratus langsdorfii) have strong direct antibacterial activity (Nayak and Nakanishi 2013). In mammals, CD4+ Tc cells have also been investigated, and they were found to function using the same cytolytic pathways as those in CD8+ Tc cells (Appay et al. 2002; Hashimoto et al. 2019; Stalder et al. 1994; Williams and Engelhard 1996). However, the precise roles of CD4+ Tc cells remain unclear. Interestingly, studies on the expression characteristics of catfish CD4 molecules showed that two CD4 genes were found on the cytotoxic cell line TS32.17, indicating that in addition to the conventional CD8+ Tc cells and CD4+ Th cells, CD4+ Tc cells may be present in teleost fish (Edholm et al. 2007). These findings show that the characteristics and functions of CD4+ cells in different fish species need to be further investigated.
CD8 is a membrane-bound extracellular receptor consisting of an αα homodimer or an αβ heterodimer (Janeway 1992; Zamoyska 1994). CD8α and CD8β consist of an IgV-like extracellular domain, a transmembrane domain and a short cytoplasmic tail, with the α and β peptides linked by disulfide bonds (Veilette et al. 1988). The heterodimer of CD8 is mostly found on mature cytotoxic T cells and thymocytes, while the homodimer is expressed on NK cells, dendritic cells (DCs) and γδ T cells (Lin et al. 1994; Terabe et al. 2008). Two of the CD8 chains have been cloned in multiple teleost species, such as rainbow trout, ginbuna crucian carp, fugu, sea bass, Atlantic salmon and flounder (Hansen and Strassburger 2000; Kato et al. 2013; Maisey et al. 2011; Moore et al. 2005; Picchietti et al. 2009; Somamoto et al. 2005; Suetake et al. 2007). CD8+ cells have been functionally identified as Tc cells in teleosts and express a heterodimer of CD8 consisting of α and β chains, as occurs in mammals (Fischer et al. 2006; Nakanishi et al. 2002, 2011; Somamoto et al. 2014a, b). In mammals, a conserved binding motif p56Lck is present in the cytoplasmic tail of CD8α, whereas it is not found in the CD8β chain. In contrast, both CD8α and CD8β genes in fish contain the binding motifs, suggesting that CD8 molecules may have signaling functions in the heterodimers and homodimers of teleosts (Quiniou et al. 2011; Tafalla et al. 2016). To date, nothing is known about the differences between homodimers and heterodimers of fish CD8 molecules and their functions as co-receptors of T cells.
T cytotoxic (Tc) cells express CD8 chains involved in the interaction with peptide-MHC class I, and Tc cells were thus identified by detecting CD8 anitgens using specific antibodies (Araki et al. 2008). Currently, several antibodies recognizing fish CD8 molecules have been generated (Table 1), and the functions of T cytotoxic cells in fish are outlined in some reviews (Fischer et al. 2006; Nakanishi et al. 2002, 2011; Somamoto et al. 2014a, b). CD8α+ leukocytes from fugu (Takifugu rubripes) were characterized by using anti-CD8α antiserum generated in mouse by DNA-immunization (Araki et al. 2008). The CD8α and CD8β genes were expressed in sorted fugu CD8α+ leukocytes, whereas CD4 and immunoglobulin light chain (IgL) genes were detected only in CD8α- cells. In addition, fugu CD8α+ leukocytes gave a response to PHA but not to LPS, suggesting that teleost CD8+ cells have characteristics similar to mammalian CD8+ T lymphocytes. In ginbuna crucian carp, it was demonstrated for the first time that CD8+ lymphocytes were the principal cells participating in specific cell-mediated cytotoxicity against allogeneic targets in fish, as reported in higher vertebrates (Toda et al. 2009). Shibasaki et al. (2010) claimed that donor-derived CD8α+ T lymphocytes in carp play critical roles in the response to acute graft-versus-host reaction as in mammals. Furthermore, the protective immunity against intracellular pathogen infection and direct antibacterial activity of CD8α+ T cells have been demonstrated in carp (Nayak and Nakanishi 2013; Yamasaki et al. 2014). After challenge with infectious salmon anaemia virus (ISAV), salmon CD8-labeled cells participated in the early activation of cellular immunity in the defense against ISAV (Hetland et al. 2010). In orange spotted grouper (Epinephelus coioides), the effector or target cells were obtained from, and the MHC class I restriction and specific cytotoxicity of CD8+ cells were measured in, the same individual fish (Chang et al. 2011). The results showed that grouper cytotoxic CD8+ cells have heterogeneous features in terms of specific antigen recognition and MHC I restriction (Chang et al. 2011). CD8α+ T cells have also been detected in trout by using mAbs and it was found that high abundances of CD8+ cells are present in the thymus, intestine and gills, but low abundances in the spleen, pronephros and blood (Takizawa et al. 2011). After stimulation with PHA, trout CD8+ cells up-regulated the Tc cells effector genes, such as perforin, granzyme and IFN-γ, which suggests the functions of teleost CD8+ T lymphocytes are similar to those in mammals (Takizawa et al. 2011). In a previous study, where flounder CD8+ lymphocytes were identified in the peripheral blood, spleen and head kidney by using an antiserum reaction to the CD8β chain, it was found that there is no cross-reaction between CD8β+ and CD4+ lymphocytes (Xing et al. 2017a). After hirame novirhabdovirus (HIRRV) infection or immunization, the ratios of CD8+ T cells increased more rapidly than CD4+ T cells, which indicates that CD8+ T cells play main roles in the response to HIRRV (Xing et al. 2018a). Similarly, Jung et al. (2021) also found that flounder CD8+ T cells were mainly involved in the adaptive immune response against viruses. The antiviral functions of CD8+ T cells in teleost fish were reviewed by Somamoto et al.(2014a, b).
It has been shown that flounder CD8+ T lymphocytes proliferate after immunization with a DNA vaccine (Xing et al. 2019a). Furthermore, studies in mammals have shown that CD8+ T lymphocytes can enhance the protective effect of vaccines after immunization with DNA plasmids (Ulmer and Otten 2000). These results suggest that the immune response of fish CD8+ T cells can also be an important evaluation indicator of the efficacy of DNA vaccines. Interestingly, mammalian CD8α can be expressed as CD8αα homodimers on different leukocytes, such as αβ or γδ T cells, NK cells, DCs, regulatory T cells and even macrophages (Addison et al. 2005; Bonneville and Lang 2002). In fugu, the expression of CD8α has also been detected in monocytes/macrophages (Araki et al. 2008). Recent studies have shown that a DC-like subpopulation co-expressing CD8α and MHC II on the surface of cells in the skin, gills, gut and olfactory organs of rainbow trout (Oncorhynchus mykiss) (Granja et al. 2015; Sepahi et al. 2016; Soleto et al. 2018, 2019). These results provide the evidence for the existence of CD8α+ DCs in non-immune tissue of teleost fish, and support the hypothesis that all mammalian cross-presenting DCs have a common origin. Due to the lack of sufficient mAbs against the CD8β chain in many fish species, it remains unknown whether CD8αα+ and CD8αβ+ lymphocyte subpopulations have different functions. Hence, further studies are needed to produce specific antibodies against both CD8α and CD8β chains in different teleost fish.
There are several other pivotal surface-associated molecules involved in the activation, proliferation and differentiation of T cells, such as CD28, cytotoxic T lymphocyte antigen 4 (CTLA-4/CD152), CD40L (CD154) and CD2. According to the mammalian paradigm, activation of T cells requires the following two sets of signals: The first signal is the delivery of processed antigen to the TCR via MHC molecules; the second is known as the costimulatory signal, which is delivered to receptors on T cells via costimulatory molecules (Hu et al. 2012; Paterson et al. 2009; Rudd et al. 2009). The co-stimulatory receptors CD28 and CTLA-4 of T cells are known to play essential roles in transmitting the second signal. CD28 and CTLA-4 have reverse effects on T cell stimulation, the activation of TCR is enhanced by CD28 but inhibited by CTLA-4 (Chen and Flies 2013). In mammals, CD28 and CTLA-4 are transmembrane protein members belonging to the IgSF, and they both interact with the same ligands, i.e., members of the B7 family, CD80 (B7-1) and CD86 (B7-2) expressed on APCs (Esensten et al. 2016; Sansom 2010). Studies have shown that both CD28 and CTLA-4 homologs are conserved in fish and the pathway of CD28-CD80/86 may present in teleosts (Bernard et al. 2006, 2007; Fang et al. 2018; González-Fernández et al. 2021; Hu et al. 2012; Huang et al. 2018; Jeswin et al. 2017; Sugamata et al. 2009; Zhang et al. 2009, 2018). The features of CD28/CTLA-4 in teleosts were discussed in depth in two reviews (Castro et al. 2011; Laing and Hansen 2011).
CD40L (also known as CD154) is a 39-kDa glycoprotein of the TNF family that is initially found on activated CD4+ T cells (Graf et al. 1992; Kooten and Banchereau 2000). The natural receptor for CD40L is the type I membrane-bound protein CD40, which was originally identified as a surface antigen expressed on mature and activated B lymphocytes (Clark 1990). In mammals, it has been shown that the CD40-CD40L mediated contact-dependent signal is essential for Th-dependent B lymphocyte proliferation, Ig production and type switching, and even memory responses (Castigli et al. 1994; Foy et al. 1993; Kawabe et al. 1994; Renshaw et al. 1994; Xu et al. 1994). CD40-CD40L interactions also play an important role in the functional communication between T lymphocytes and DCs (Caux et al. 1994; Cella et al. 1996). Furthermore, in mammals, CD40L-CD40 interactions direct T lymphocyte maturation towards the Th1 phenotype through the induction of proinflammatory cytokines (Mackey et al. 1998; Pinchuk et al. 1994). In fish, CD40 and CD40L genes were cloned in various of fish species, such as flounder (Paralichthys olivaceus), trout (Oncorhynchus mykiss), zebrafish (Danio rerio), Atlantic salmon (Salmo salar) and humphead snapper (Lutjanus sanguineus) (Cai et al. 2017; Glenney and Wiens 2007; Gong et al. 2009; Lagos et al. 2012; Park et al. 2005). In zebrafish, the expression of CD40L was inhibited by cyclosporin A, and the production of IgM was affected by the supplement of anti-CD40L or soluble CD40 (Gong et al. 2009). These results provide evidence for the existence of a CD40-CD40L mediated costimulatory pathway in fish. Interestingly, Xing et al. (2018b) showed that CD40 was not only expressed on sIgM+ B lymphocytes, but also on CD4+ and CD8+ T subsets. In mammals, increasing evidence confirms the presence of CD40 molecules on T cells, and CD40 may act on both CD4 and CD8 T lymphocytes (Munroe and Bishop 2007; Munroe 2009). However, further studies are needed to elucidate the exact role of CD40 in fish T cells.
CD2 (lymphocyte function-associated antigen-2) is a cell adhesion molecule expressed on all mature peripheral blood T cells, thymocytes and natural killer (NK) cells (Davis and van der Merwe 1996; Seed and Aruffo 1987; Springer et al. 1987). Its ligand, CD58, which is also an adhesion molecule, is expressed on hemopoietic and non-hemopoietic lineages such as DCs, macrophages, erythrocytes, and endothelial cells (Dengler et al. 1992; Karmann et al. 1996; Moingeon et al. 1989; Ocklind et al. 1992). Functionally, the interaction of CD58 with CD2 plays an important role in the adhesion between the T cells and APCs and also provides the optimal spacing for the antigen recognition of TCR (Cheadle et al. 2012; Zhu et al. 2006). CD2 was identified in channel catfish and zebrafish, and the interaction of CD58 with CD2 has been well demonstrated in zebrafish where it provides a primary costimulatory signal for the complete activation of CD4+ T cells in adaptive humoral immunity (Shao et al. 2018; Taylor et al. 2015).
In conclusion, a variety of CD molecules exist on T cells in fish, and in this review, we described the role of CD molecules as surface markers in the identification of teleost T lymphocyte subpopulations. It is undeniable that some CD antigens, which are the surface markers of T cells, are also expressed on other cell lineages in fish. At present, identification and characterization of CD molecules on fish T cells are far from sufficient, and antibodies for the clear discrimination of Th and Tc subsets according to the CD antigens of mammals are urgently needed for multiple fish species. There is a lack of direct evidence about the precise role of CD molecules in T cell activation and signaling from fish, and more studies are needed to support these hypotheses. In addition, the effectors of Tc (perforin and granzyme) should be well characterized, which can give insights into the killing mechanism of CD8+ Tc in fish. The definition of different phenotypical CD4+ Th subsets in fish still needs more evidences, and multiple cytokines and transcription factors involved in Th-type immunity should be functionally characterized. Furthermore, the mechanisms by which fish CD4+ Th cells activate B cells, CD8+ T cells and macrophages need to be elucidated. The antibodies against critical cytokines, such as IFN-γ, IL-2, IL-4 and IL-17, should be produced as they will be powerful tools to detect the preference of cytokine secretion by CD4+ T cells, and meaningful for the differentiation of CD4+ T cells in fish. Thus, there is still an urgent need to accurately delineate the pathways of T cell response to vaccination as well as how the fish T cell subsets are able to regulate a protective response. In combination, such knowledge would deepen our understanding about the role of fish T lymphocyte subsets in adaptive immunity and facilitate the health management and development of vaccines in aquaculture.
This study was supported by the National Key Research and Development Program of China (2018YFD0900503), the National Natural Science Foundation of China (31730101; 32173005; 31672684; 31672685), Shandong Provincial Natural Science Foundation (ZR2020KC025).
H-FT and JX contributed to the conception and drafted the manuscript. X-QT, HC, X-ZS and W-BZ revised the manuscript. All the authors read and approved this version of the final manuscript.
All data generated or analyzed during this study are included in the manuscript.
The authors declare that they have no conflicts of interests.
This article does not contain any studies with human participants or animals performed by any of the authors.
This article is licensed under a Creative Commons Attribution 4.0 International License, which permits use, sharing, adaptation, distribution and reproduction in any medium or format, as long as you give appropriate credit to the original author(s) and the source, provide a link to the Creative Commons licence, and indicate if changes were made. The images or other third party material in this article are included in the article's Creative Commons licence, unless indicated otherwise in a credit line to the material. If material is not included in the article's Creative Commons licence and your intended use is not permitted by statutory regulation or exceeds the permitted use, you will need to obtain permission directly from the copyright holder. To view a copy of this licence, visit http://creativecommons.org/licenses/by/4.0/.
Addison EG, North J, Bakhsh I, Marden C, Haq S, Al-Sarraj S, Malayeri R, Wickremasinghe RG, Davies JK, Lowdell MW (2005) Ligation of CD8alpha on human natural killer cells prevents activation-induced apoptosis and enhances cytolytic activity. Immunology 116: 354-361 doi: 10.1111/j.1365-2567.2005.02235.x
|
Appay V, Zaunders JJ, Papagno L, Sutton J, Jaramillo A, Waters A, Easterbrook P, Grey P, Smith D, McMichael AJ, Cooper DA, Rowland-Jones SL, Kelleher AD (2002) Characterization of CD4+ CTLs ex vivo. J Immunol 168: 5954-5958 doi: 10.4049/jimmunol.168.11.5954
|
Araki K, Suetake H, Kikuchi K, Suzuki Y (2005) Characterization and expression analysis of CD3ɛ and CD3γ/δ in fugu, Takifugu rubripes. Immunogenetics 57: 158-163 doi: 10.1007/s00251-005-0772-8
|
Araki K, Akatsu K, Suetake H, Kikuchi K, Suzuki Y (2008) Characterization of CD8+ leukocytes in fugu (Takifugu rubripes) with antiserum against fugu CD8α. Dev Comp Immunol 32: 850-858 doi: 10.1016/j.dci.2007.12.005
|
Ashfaq H, Soliman H, Saleh M, El-Matbouli M (2019) CD4: a vital player in the teleost fish immune system. Vet Res 50: 1-11 doi: 10.1186/s13567-018-0620-0
|
Ashfaq H, El-Matbouli M, Soliman H (2020) Identification and molecular characterization of CD4 genes in brown trout (Salmo trutta). Dev Comp Immunol 107: 103663 doi: 10.1016/j.dci.2020.103663
|
Ashwell JD, Klausner RD (1990) Genetic and mutational analysis of the T cell antigen receptor. Annu Rev Immunol 8: 139-167 doi: 10.1146/annurev.iy.08.040190.001035
|
Baixeras E, Huard B, Miossec C, Jitsukawa S, Martin M, Hercend T, Auffray C, Triebel F, Piatier-Tonneau D (1992) Characterization of the lymphocyte activation gene 3-encoded protein. A new ligand for human leukocyte antigen class II antigens. J Exp Med 176: 327-337 doi: 10.1084/jem.176.2.327
|
Bernard A, Boumsell L (1984) The clusters of differentiation (CD) defined by the First International Workshop on human leucocyte differentiation antigens. Hum Immunol 11: 1-10 doi: 10.1016/0198-8859(84)90051-X
|
Bernard D, Riteau B, Hansen JD, Phillips RB, Michel F, Boudinot P, Benmansour A (2006) Costimulatory receptors in a teleost fish: typical CD28, elusive CTLA4. J Immunol 176: 4191-4200 doi: 10.4049/jimmunol.176.7.4191
|
Bernard D, Hansen JD, Pasquier LD, Lefranc MP, Benmansour A, Boudinot P (2007) Costimulatory receptors in jawed vertebrates: conserved CD28, odd CTLA4 and multiple BTLAs. Dev Comp Immunol 31: 255-271 doi: 10.1016/j.dci.2006.06.003
|
Bertram EM, Wilkinson RG, Lee BA, Jilbert AR, Kotlarski I (1996) Identification of duck T lymphocytes using an anti-human T cell (CD3) antiserum. Vet Immunol Immunopathol 51: 353-363 doi: 10.1016/0165-2427(95)05528-2
|
Boardman T, Warner C, Ramirez-Gomez F, Matrisciano J, Bromage E (2012) Characterization of an anti-rainbow trout (Oncorhynchus mykiss) CD3ε monoclonal antibody. Vet Immunol Immunopathol 145: 511-515 doi: 10.1016/j.vetimm.2011.11.017
|
Bonneville M, Lang F (2002) CD8: from coreceptor to comodulator. Nat Immunol 3: 12-14 doi: 10.1038/ni0102-12
|
Boumsell L (1996) The international workshops and conferences on human leukocyte differentiation antigens. Birth, current status and future. Tissue Antigens 48: 238-241 doi: 10.1111/j.1399-0039.1996.tb02641.x
|
Buonocore F, Randelli E, Casani D, Guerra L, Picchietti S, Costantini S, Facchiano AM, Zou J, Secombes CJ, Scapigliati G (2008) A CD4 homologue in sea bass (Dicentrarchus labrax): molecular characterization and structural analysis. Mol Immunol 45: 3168-3177 doi: 10.1016/j.molimm.2008.02.024
|
Cai J, Fan Y, Xia H, Lu Y, Jian J, Wu Z (2017) Identification and characterization of CD40 from humphead snapper (Lutjanus sanguineus). Fish Shellfish Immunol 70: 665-672 doi: 10.1016/j.fsi.2017.09.058
|
Castigli E, Alt FW, Davidson L, Bottaro A, Mizoguchi E, Bhan AK, Geha RS (1994) CD40-deficient mice generated by recombination-activating gene-2-deficient blastocyst complementation. Proc Natl Acad Sci USA 91: 12135-12139 doi: 10.1073/pnas.91.25.12135
|
Castro R, Bernard D, Lefranc MP, Six A, Benmansour A, Boudinot P (2011) T cell diversity and TCR repertoires in teleost fish. Fish Shellfish Immunol 31: 644-654 doi: 10.1016/j.fsi.2010.08.016
|
Caux C, Massacrier C, Vanbervliet B, Dubois B, Van Kooten C, Durand I, Banchereau J (1994) Activation of human dendritic cells through CD40 crosslinking. J Exp Med 180: 1263-1272 doi: 10.1084/jem.180.4.1263
|
Cella M, Scheidegger D, Palmer-Lehmann K, Lane P, Lanzavecchia A, Alber G (1996) Ligation of CD40 on dendritic cells triggers production of high levels of interleukin-12 and enhances T cell stimulatory capacity: T-T help via APC activation. J Exp Med 184: 747-752 doi: 10.1084/jem.184.2.747
|
Chan MM, Chen CL, Ager LL, Cooper MD (1988) Identification of the avian homologues of mammalian CD4 and CD8 antigens. J Immunol 140: 2133-2138
|
Chang Y, Kai Y, Chi S, Song Y (2011) Cytotoxic CD8α+ leukocytes have heterogeneous features in antigen recognition and class I MHC restriction in grouper. Fish Shellfish Immunol 30: 1283-1293 doi: 10.1016/j.fsi.2011.03.018
|
Cheadle EJ, Rothwell DG, Bridgeman JS, Sheard VE, Hawkins RE, Gilham DE (2012) Ligation of the CD2 co-stimulatory receptor enhances IL-2 production from first generation chimeric antigen receptor T cells. Gene Ther 19: 1114-1120 doi: 10.1038/gt.2011.192
|
Chen L, Flies DB (2013) Molecular mechanisms of T cell co-stimulation and co-inhibition. Nat Rev Immunol 13: 227-242 doi: 10.1038/nri3405
|
Cho DH, Bae JS, Jeong JM, Han HJ, Lee DC, Cho MY, Jung SH, Kim DH, Park CI (2017) The first report of CD2 associated protein gene, in a teleost (Rock bream, Oplegnathus fasciatus): an investigation of the immune response upon infection with several pathogens. Fish Shellfish Immunol 67: 1-6 doi: 10.1016/j.fsi.2017.05.045
|
Clark EA (1990) CD40: a cytokine receptor in search of a ligand. Tissue Antigens 36: 33-36 doi: 10.1111/j.1399-0039.1990.tb01795.x
|
Cook MT, Morrison RN, Wilkinson R, Nowak BF, Hayball PJ, Hayball JD (2001) A screen of mammalian antibodies on snapper (Pagrus auratus, Sparidae) peripheral blood leukocytes reveals cross reactivity of an anti-human CD3 antibody with a population of mIg- cells. Dev Comp Immunol 25: 553-559 doi: 10.1016/S0145-305X(01)00024-6
|
Cruse JM, Lewis RE, Wang H, Schreuder G, Marsh S, Kennedy LJ (2004) Cluster of differentiation (CD) antigens. In: Cruse JM, Lewis RE, Wang H (eds) Immunology guidebook. Academic Press, San Diego, pp 47-124
|
Davis SJ, van der Merwe PA (1996) The structure and ligand interactions of CD2: implications for T-cell function. Immunol Today 17: 177-187 doi: 10.1016/0167-5699(96)80617-7
|
Dengler TJ, Hoffmann JC, Knolle P, Albert-Wolf M, Roux M, Wallich R, Meuer SC (1992) Structural and functional epitopes of the human adhesion receptor CD58 (LFA-3). Eur J Immunol 22: 2809-2817 doi: 10.1002/eji.1830221109
|
Edholm ES, Stafford JL, Quiniou SM, Waldbieser G, Miller NW, Bengtén E, Wilson M (2007) Channel catfish, Ictalurus punctatus, CD4-like molecules. Dev Comp Immunol 31: 172-187 doi: 10.1016/j.dci.2006.05.012
|
Engel P, Boumsell L, Balderas R, Bensussan A, Gattei V, Horejsi V, Jin B, Malavasi F, Mortari F, Schwartz-Albiez R, Stockinger H, van Zelm MC, Zola H, Clark G (2015) CD nomenclature 2015: human leukocyte differentiation antigen workshops as a driving force in immunology. J Immunol 195: 4555-4563 doi: 10.4049/jimmunol.1502033
|
Esensten JH, Helou YA, Chopra G, Weiss A, Bluestone JA (2016) CD28 costimulation: from mechanism to therapy. Immunity 44: 973-988 doi: 10.1016/j.immuni.2016.04.020
|
Fang D, Zhao C, Jiang S, Zhou Y, Xu D (2018) Toxic function of CD28 involving in the TLR/MyD88 signal pathway in the river pufferfish (Takifugu obscurus) after exposed to tributyltin chloride (TBT-Cl). Gene 688: 84-92
|
Fischer U, Utke K, Somamoto T, Köllner B, Ototake M, Nakanishi T (2006) Cytotoxic activities of fish leucocytes. Fish Shellfish Immunol 20: 209-226 doi: 10.1016/j.fsi.2005.03.013
|
Fischer U, Koppang EO, Nakanishi T (2013) Teleost T and NK cell immunity. Fish Shellfish Immunol 35: 197-206 doi: 10.1016/j.fsi.2013.04.018
|
Foy TM, Shepherd DM, Durie FH, Aruffo A, Ledbetter JA, Noelle RJ (1993) In vivo CD40-gp39 interactions are essential for thymus-dependent humoral immunity, II: Prolonged suppression of the humoral immune response by an antibody to the ligand for CD40, gp39. J Exp Med 178: 1567-1575 doi: 10.1084/jem.178.5.1567
|
Glenney GW, Wiens GD (2007) Early diversification of the TNF superfamily in teleosts: genomic characterization and expression analysis. J Immunol 178: 7955-7973 doi: 10.4049/jimmunol.178.12.7955
|
Göbel T, Dangy JP (2000) Evidence for a stepwise evolution of the CD3 family. J Immunol 164: 879-883 doi: 10.4049/jimmunol.164.2.879
|
Gong Y, Xiang L, Shao J (2009) CD154-CD40 interactions are essential for thymus-dependent antibody production in zebrafish: insights into the origin of costimulatory pathway in helper T cell-regulated adaptive immunity in early vertebrates. J Immunol 182: 7749-7762 doi: 10.4049/jimmunol.0804370
|
González-Fernández C, Esteban MA, Cuesta A (2021) Molecular characterization of the T cell costimulatory receptors CD28 and CTLA4 in the European sea bass. Fish Shellfish Immunol 109: 106-115 doi: 10.1016/j.fsi.2020.12.006
|
Gordon S, Taylor PR (2005) Monocyte and macrophage heterogeneity. Nat Rev Immunol 5: 953-964 doi: 10.1038/nri1733
|
Graf D, Korthauer U, Mages HW, Senger G, Kroczek RA (1992) Cloning of TRAP, a ligand for CD40 on human T cells. Eur J Immunol 22: 3191-3194 doi: 10.1002/eji.1830221226
|
Granja AG, Leal E, Pignatelli J, Castro R, Abos B, Kato G, Fischer U, Tafalla C (2015) Identification of teleost skin CD8α+ dendritic-like cells, representing a potential common ancestor for mammalian cross-presenting dendritic cells. J Immunol 195: 1825-1837 doi: 10.4049/jimmunol.1500322
|
Hansen JD, Strassburger P (2000) Description of an ectothermic TCR coreceptor, CD8α, in rainbow trout. J Immunol 164: 3132-3139 doi: 10.4049/jimmunol.164.6.3132
|
Hashimoto K, Kouno T, Ikawa T, Hayatsu N, Carninci P (2019) Single-cell transcriptomics reveals expansion of cytotoxic CD4 T-cells in supercentenarians. Proc Natl Acad Sci USA 116: 201907883
|
Hayday AC (2000) γδ cells: a right time and a right place for a conserved third way of protection. Annu Rev Immunol 18: 975-1026 doi: 10.1146/annurev.immunol.18.1.975
|
Hetland DL, Jorgensen SM, Skjodt K, Dale OB, Falk K, Xu C, Mikalsen AB, Grimholt U, Gjoen T, Press CM (2010) In situ localisation of major histocompatibility complex class I and class II and CD8 positive cells in infectious salmon anaemia virus (ISAV)-infected Atlantic salmon. Fish Shellfish Immunol 28: 30-39 doi: 10.1016/j.fsi.2009.09.011
|
Hu Y, Sun B, Tian D, Sun L (2012) Molecular characterization of Cynoglossus semilaevis CD28. Fish Shellfish Immunol 32: 934-938 doi: 10.1016/j.fsi.2012.02.021
|
Huang Y, Wang Z, Zheng Q, Tang J, Cai J, Lu Y, Jian J (2018) Conservation of structural and interactional features of CD28 and CD80/86 molecules from Nile tilapia (Oreochromis niloticus). Fish Shellfish Immunol 72: 95-103 doi: 10.1016/j.fsi.2017.10.008
|
Janeway CA Jr (1992) The T cell receptor as a multicomponent signalling machine: CD4/CD8 coreceptors and CD45 in T cell activation. Annu Rev Immunol 10: 645-674 doi: 10.1146/annurev.iy.10.040192.003241
|
Jeswin J, Jeong SM, Jeong JM, Bae JS, Kim MC, Kim DH, Park CI (2017) Molecular characterization of a T cell costimulatory receptor, CD28 of rock bream (Oplegnathus fasciatus): transcriptional expression during bacterial and viral stimulation. Fish Shellfish Immunol 66: 354-359 doi: 10.1016/j.fsi.2017.05.013
|
Jung JW, Lee JS, Kim YR, Im SP, Kim SW, Lazarte JMS, Kim J, Thompson KD, Suh JP, Jung TS (2017) Development of a monoclonal antibody against the CD3ε of olive flounder (Paralichthys olivaceus) and its application in evaluating immune response related to CD3ε. Fish Shellfish Immunol 65: 179-185 doi: 10.1016/j.fsi.2017.04.016
|
Jung JW, Lee JS, Kim J, Im SP, Kim SW, Lazarte JMS, Kim YR, Chun JH, Ha MW, Kim NN, Thompson KD, Kim HJ, Jung TS (2020a) Involvement of CD4-1 T cells in the cellular immune response of olive flounder (Paralichthys olivaceus) against viral hemorrhagic septicemia virus (VHSV) and nervous necrosis virus (NNV) infection. Dev Comp Immunol 103: 103518 doi: 10.1016/j.dci.2019.103518
|
Jung JW, Chun JH, Lee JS, Kim SW, Lee AR, Kim J, Lazarte JMS, Kim YR, Kim HJ, Thompson KD, Jung TS (2020b) Characterization of CD4-positive lymphocytes in the antiviral response of olive flounder (Paralichthys oliveceus) to nervous necrosis virus. Int J Mol Sci 21: 4180 doi: 10.3390/ijms21114180
|
Jung JW, Lee AR, Kim J, Kim YR, Lazarte JMS, Lee JS, Thompson KD, Kim H, Jung TS (2021) Elucidating the functional roles of helper and cytotoxic T cells in the cell-mediated immune responses of olive flounder (Paralichthys olivaceus). Int J Mol Sci 22: 847 doi: 10.3390/ijms22020847
|
Karmann K, Hughes CC, Fanslow WC, Pober JS (1996) Endothelial cells augment the expression of CD40 ligand on newly activated human CD4+ T cells through a CD2/LFA-3 signaling pathway. Eur J Immunol 26: 610-617 doi: 10.1002/eji.1830260316
|
Kato G, Goto K, Akune I, Aoka S, Kondo H, Hirono I (2013) CD4 and CD8 homologues in Japanese flounder, Paralichthys olivaceus: differences in the expressions and localizations of CD4-1, CD4-2, CD8α and CD8β. Dev Comp Immunol 39: 293-301 doi: 10.1016/j.dci.2012.09.004
|
Kato G, Kakazu T, Yamada M, Lau L, Nakajima K, Sato S, Nakanishi T, Endo M, Sano M (2019) Granulomatous inflammation in ginbuna crucian carp Carassius auratus langsdorfii against Mycobacterium gordonae. Dev Comp Immunol 91: 93-100 doi: 10.1016/j.dci.2018.10.009
|
Kawabe T, Naka T, Yoshida K, Tanaka T, Fujiwara H, Suematsu S, Yoshida N, Kishimoto T, Kikutani H (1994) The immune responses in CD40-deficient mice: impaired immunoglobulin class switching and germinal center formation. Immunity 1: 167-178 doi: 10.1016/1074-7613(94)90095-7
|
Keresztes G, Glavits R, Krenacs L, Kurucz E, Ando I (1996) An anti-CD3 epsilon serum detects T lymphocytes in paraffin-embedded pathological tissues in many animal species. Immunol Lett 50: 167-172 doi: 10.1016/0165-2478(96)02534-5
|
Kim MC, Park CI (2005) Cloning and characterization of two distinct CD3 genes from olive flounder Paralichthys olivaceus. J Fish Sci Technol 8: 56-64
|
Kim PW, Sun ZY, Blacklow SC, Wagner G, Eck MJ (2003) A zinc clasp structure tethers Lck to T cell coreceptors CD4 and CD8. Science 301: 1725-1728 doi: 10.1126/science.1085643
|
Klausner RD, Lippincott-Schwartz J, Bonifacino JS (1990) The T cell antigen receptor: insights into organelle biology. Annu Rev Cell Biol 1990: 403-431
|
Kono T, Korenaga H (2013) Cytokine gene expression in CD4 positive cells of the Japanese Pufferfish Takifugu Rubripes. PLoS ONE 8: e66364 doi: 10.1371/journal.pone.0066364
|
Kooten C, Banchereau J (2000) CD40-CD40 ligand. J Leukoc Biol 67: 2-17 doi: 10.1002/jlb.67.1.2
|
Koppang EO, Fischer U, Moore L, Tranulis MA, Dijkstra JM, Kollner B, Aune L, Jirillo E, Hordvik I (2010) Salmonid T cells assemble in the thymus, spleen and in novel interbranchial lymphoid tissue. J Anat 217: 728-739 doi: 10.1111/j.1469-7580.2010.01305.x
|
Kuhns MS, Davis MM, Garcia KC (2006) Deconstructing the form and function of the TCR/CD3 complex. Immunity 24: 133-139 doi: 10.1016/j.immuni.2006.01.006
|
Lagos LX, Iliev DB, Helland R, Rosemblatt M, Jørgensen JB (2012) CD40L-a costimulatory molecule involved in the maturation of antigen presenting cells in Atlantic salmon (Salmo salar). Dev Comp Immunol 38: 416-430 doi: 10.1016/j.dci.2012.07.011
|
Laing KJ, Hansen JD (2011) Fish T cells: recent advances through genomics. Dev Comp Immunol 35: 1282-1295 doi: 10.1016/j.dci.2011.03.004
|
Laing KJ, Zou JJ, Purcell MK, Phillips R, Secombes CJ, Hansen JD (2006) Evolution of the CD4 family: teleost fish possess two divergent forms of CD4 in addition to lymphocyte activation gene-3. J Immunol 177: 3939-3951 doi: 10.4049/jimmunol.177.6.3939
|
Langenau DM, Zon LI (2005) The zebrafish: a new model of T-cell and thymic development. Nat Rev Immunol 5: 307-317 doi: 10.1038/nri1590
|
Lin T, Matsuzaki G, Yoshida H, Kobayashi N, Kenai H, Omoto K, Nomoto K (1994) CD3-CD8+ intestinal intraepithelial lymphocytes (IEL) and the extrathymic development of IEL. Eur J Immunol 24: 1080-1087 doi: 10.1002/eji.1830240511
|
Liu Y, Moore L, Koppang EO, Hordvik I (2008) Characterization of the CD3ζ, CD3γδ and CD3ε subunits of the T cell receptor complex in Atlantic salmon. Dev Comp Immunol 32: 26-35 doi: 10.1016/j.dci.2007.03.015
|
Mackey MF, Barth RJ, Noelle RJ (1998) The role of CD40-CD154 interactions in the priming, differentiation and effector function of helper and cytotoxic T cells. J Leukocyte Biol 63: 418-428 doi: 10.1002/jlb.63.4.418
|
Maisey K, Toro-Ascuy D, Montero R, Reyes-López FE, Imarai M (2011) Identification of CD3ε, CD4, CD8β splice variants of Atlantic salmon. Fish Shellfish Immunol 31: 815-822
|
Maisey K, Montero R, Corripio-Miyar Y, Toro-Ascuy D, Valenzuela B, Reyes-Cerpa S, Sandino AM, Zou J, Wang T, Secombes CJ, Imarai M (2016) Isolation and characterization of salmonid CD4+ T cells. J Immunol 196: 4150-4163 doi: 10.4049/jimmunol.1500439
|
Malmstrøm M, Matschiner M, Tørresen OK, Star B, Snipen LG, Hansen TF, Baalsrud HT, Nederbragt AJ, Hanel R, Salzburger W, Stenseth NC, Jakobsen KS, Jentoft S (2016) Evolution of the immune system influences speciation rates in teleost fishes. Nat Genet 48: 1204-1210 doi: 10.1038/ng.3645
|
Mao K, Chen W, Mu Y, Ao J, Chen X (2017) Molecular characterization and expression analysis during embryo development of CD4-1 homologue in large yellow croaker Larimichthys crocea. Fish Shellfish Immunol 64: 146-154 doi: 10.1016/j.fsi.2017.02.044
|
Merwe PA, Davis SJ (2002) Molecular interactions mediating T cell antigen recognition. Annu Rev Immunol 21: 659-684
|
Moingeon P, Chang HC, Wallner BP, Stebbins C, Frey AZ, Reinherz EL (1989) CD2-mediated adhesion facilitates T lymphocyte antigen recognition function. Nature 339: 312-314 doi: 10.1038/339312a0
|
Moore LJ, Somamoto T, Lie KK, Dijkstra JM, Hordvik I (2005) Characterisation of salmon and trout CD8α and CD8β. Mol Immunol 42: 1225-1234 doi: 10.1016/j.molimm.2004.11.017
|
Moore LJ, Dijkstra JM, Koppang EO, Hordvik I (2009) CD4 homologues in Atlantic salmon. Fish Shellfish Immunol 26: 10-18 doi: 10.1016/j.fsi.2008.09.019
|
Munroe ME (2009) Functional roles for T cell CD40 in infection and autoimmune disease: the role of CD40 in lymphocyte homeostasis. Semin Immunol 21: 283-288 doi: 10.1016/j.smim.2009.05.008
|
Munroe ME, Bishop GA (2007) A costimulatory function for T cell CD40. J Immunol 178: 671-682 doi: 10.4049/jimmunol.178.2.671
|
Nakanishi T, Fischer U, Dijkstra JM, Hasegawa S, Somamoto T, Okamoto N, Ototake M (2002) Cytotoxic T cell function in fish. Dev Comp Immunol 26: 131-139 doi: 10.1016/S0145-305X(01)00055-6
|
Nakanishi T, Toda H, Shibasaki Y, Somamoto T (2011) Cytotoxic T cells in teleost fish. Dev Comp Immunol 35: 1317-1323 doi: 10.1016/j.dci.2011.03.033
|
Nakanishi T, Shibasaki Y, Matsuura Y (2015) T cells in fish. Biology 4: 640-663 doi: 10.3390/biology4040640
|
Nayak SK, Nakanishi T (2013) Direct antibacterial activity of CD8+/CD4+ T-cells in ginbuna crucian carp, Carassius auratus langsdorfii. Fish Shellfish Immunol 34: 136-141 doi: 10.1016/j.fsi.2012.10.016
|
Ocklind G, Friedrichs D, Peters JH (1992) Expression of CD54, CD58, CD14, and HLA-DR on macrophages and macrophage-derived accessory cells and their accessory capacity. Immunol Lett 31: 253-258 doi: 10.1016/0165-2478(92)90123-6
|
Pancer Z, Mayer WE, Klein J, Cooper MD (2004) Prototypic T cell receptor and CD4-like coreceptor are expressed by lymphocytes in the agnathan sea lamprey. Proc Natl Acad Sci USA 101: 13273-13278 doi: 10.1073/pnas.0405529101
|
Park CI, Hirono I, Enomoto J, Nam BH, Aoki T (2001) Cloning of Japanese flounder Paralichthys olivaceus CD3 cDNA and gene, and analysis of its expression. Immunogenetics 53: 130-135 doi: 10.1007/s002510100311
|
Park CI, Hirono I, Hwang JY, Aoki T (2005) Characterization and expression of a CD40 homolog gene in Japanese flounder Paralichthys olivaceus. Immunogenetics 57: 682-689 doi: 10.1007/s00251-005-0032-y
|
Patel S, Øvergard AC, Nerland AH (2009) A CD4 homologue in Atlantic halibut (Hippoglossus hippoglossus): molecular cloning and characterisation. Fish Shellfish Immunol 26: 377-384 doi: 10.1016/j.fsi.2008.11.013
|
Paterson AM, Vanguri VK, Sharpe AH (2009) SnapShot: B7/CD28 costimulation. Cell 137: 974 doi: 10.1016/j.cell.2009.05.015
|
Picchietti S, Guerra L, Selleri L, Buonocore F, Abelli L, Scapigliati G, Mazzini M, Fausto AM (2008) Compartmentalisation of T cells expressing CD8α and TCRβ in developing thymus of sea bass Dicentrarchus labrax (L. ). Dev Comp Immunol 32: 92-99 doi: 10.1016/j.dci.2007.04.002
|
Picchietti S, Guerra L, Buonocore F, Randelli E, Fausto AM, Abelli L (2009) Lymphocyte differentiation in sea bass thymus: CD4 and CD8-alpha gene expression studies. Fish Shellfish Immunol 27: 50-56 doi: 10.1016/j.fsi.2009.04.003
|
Pinchuk LM, Polacino PS, Agy MB, Klaus SJ, Clark EA (1994) The role of CD40 and CD80 accessory cell molecules in dendritic cell-dependent HIV-1 infection. Immunity 1: 317-325 doi: 10.1016/1074-7613(94)90083-3
|
Prinz I, Silva-Santos B, Pennington DJ (2013) Functional development of γδ T cells. Eur J Immunol 43: 1988-1994 doi: 10.1002/eji.201343759
|
Quiniou SM, Sahoo M, Edholm ES, Bengten E, Wilson M (2011) Channel catfish CD8alpha and CD8beta co-receptors: characterization, expression and polymorphism. Fish Shellfish Immunol 30: 894-901 doi: 10.1016/j.fsi.2011.01.011
|
Randelli E, Scapigliati G, Buonocore F (2011) CD3 γ/δ in sea bass (Dicentrarchus labrax): molecular characterization and expression analysis. Res Immunol 1: 31-35 doi: 10.1016/j.rinim.2011.08.003
|
Renshaw BR, Fanslow WC, Armitage RJ, Campbell KA, Liggitt D, Wright B, Davison BL, Maliszewski CR (1994) Humoral immune responses in CD40 ligand-deficient mice. J Exp Med 180: 1889-1900 doi: 10.1084/jem.180.5.1889
|
Romano N, Caccia E, Piergentili R, Rossi F, Ficca AG, Ceccariglia S, Mastrolia L (2011) Antigen-dependent T lymphocytes (TcRβ+) are primarily differentiated in the thymus rather than in other lymphoid tissues in sea bass (Dicentrarchus labrax, L. ). Fish Shellfish Immunol 30: 773-782 doi: 10.1016/j.fsi.2010.12.032
|
Rombout JH, Joosten PH, Engelsma MY, Vos AP, Taverne N, Taverne-Thiele JJ (1998) Indications for a distinct putative T cell population in mucosal tissue of carp (Cyprinus carpio L. ). Dev Comp Immunol 22: 63-77 doi: 10.1016/S0145-305X(97)00048-7
|
Rudd CE, Taylor A, Schneider H (2009) CD28 and CTLA-4 coreceptor expression and signal transduction. Immunol Rev 229: 12-26 doi: 10.1111/j.1600-065X.2009.00770.x
|
Salmond RJ, Filby A, Qureshi I, Caserta S, Zamoyska R (2010) T-cell receptor proximal signaling via the Src-family kinases, Lck and Fyn, influences T-cell activation, differentiation, and tolerance. Immunol Rev 228: 9-22
|
Sansom DM (2010) CD28, CTLA-4 and their ligands: who does what and to whom? Immunology 101: 169-177
|
Scapigliati G, Romano N, Abelli L, Meloni S, Ficca AG, Buonocore F, Bird S, Secombes CJ (2000) Immunopurification of T-cells from sea bass Dicentrarchus labrax (L. ). Fish Shellfish Immunol 10: 329-341 doi: 10.1006/fsim.1999.0243
|
Seed B, Aruffo A (1987) Molecular cloning of the CD2 antigen, the T-cell erythrocyte receptor, by a rapid immunoselection procedure. Proc Natl Acad Sci USA 84: 3365-3369 doi: 10.1073/pnas.84.10.3365
|
Sepahi A, Casadei E, Tacchi L, Munoz P, LaPatra SE, Salinas I (2016) Tissue microenvironments in the nasal epithelium of rainbow trout (Oncorhynchus mykiss) define two distinct CD8α+ cell populations and establish regional immunity. J Immunol 197: 4453-4463 doi: 10.4049/jimmunol.1600678
|
Shang N, Sun X, Hu W, Wang Y, Guo Q (2008) Molecular cloning and characterization of common carp (Cyprinus carpio L. ) TCRγ and CD3γ/δ chains. Fish Shellfish Immunol 24: 412-425 doi: 10.1016/j.fsi.2007.12.007
|
Shao T, Shi W, Zheng J, Xu X, Lin A, Xiang L, Shao J (2018) Costimulatory function of CD58/CD2 interaction in adaptive humoral immunity in a zebrafish model. Front Immunol 9: 1204 doi: 10.3389/fimmu.2018.01204
|
Shibasaki Y, Toda H, Kobayashi I, Moritomo T, Nakanishi T (2010) Kinetics of CD4+ and CD8α+ T-cell subsets in graft-versus-host reaction (GVHR) in ginbuna crucian carp Carassius auratus langsdorfii. Dev Comp Immunol 34: 1075-1081 doi: 10.1016/j.dci.2010.05.009
|
Silva-Santos B, Serre K, Norell H (2015) γδ T cells in cancer. Nat Rev Immunol 15: 683-691 doi: 10.1038/nri3904
|
Soleto I, Fischer U, Tafalla C, Granja AG (2018) Identification of a potential common ancestor for mammalian cross-presenting dendritic cells in teleost respiratory surfaces. Front Immunol 9: 59 doi: 10.3389/fimmu.2018.00059
|
Soleto I, Granja AG, Simón R, Morel E, Díaz-Rosales P, Tafalla C (2019) Identification of CD8α+ dendritic cells in rainbow trout (Oncorhynchus mykiss) intestine. Fish Shellfish Immunol 89: 309-318 doi: 10.1016/j.fsi.2019.04.001
|
Somamoto T, Yoshiura Y, Nakanishi T, Ototake M (2005) Molecular cloning and characterization of two types of CD8 alpha from ginbuna crucian carp, Carassius auratus langsdorfii. Dev Comp Immunol 29: 693-702 doi: 10.1016/j.dci.2004.11.006
|
Somamoto T, Kondo M, Nakanishi T, Nakao M (2014a) Helper function of CD4+ lymphocytes in antiviral immunity in ginbuna crucian carp, Carassius auratus langsdorfii. Dev Comp Immunol 44: 111-115 doi: 10.1016/j.dci.2013.12.008
|
Somamoto T, Koppang EO, Fischer U (2014b) Antiviral functions of CD8+ cytotoxic T cells in teleost fish. Dev Comp Immunol 43: 197-204 doi: 10.1016/j.dci.2013.07.014
|
Springer TA, Dustin ML, Kishimoto TK, Marlin SD (1987) The lymphocyte function-associated LFA-1, CD2, and LFA-3 molecules: cell adhesion receptors of the immune system. Annu Rev Immunol 5: 223-252 doi: 10.1146/annurev.iy.05.040187.001255
|
Stalder T, Hahn S, Erb P (1994) Fas antigen is the major target molecule for CD4+ T cell-mediated cytotoxicity. J Immunol 152: 1127-1133
|
Star B, Nederbragt AJ, Jentoft S, Grimholt U, Malmstram M, Gregers TF, Rounge TB, Paulsen J, Solbakken MH, Sharma A, Wetten OF, Lanzén A, Winer R, Knight J, Vogel J, Aken B, Andersen Ø, Lagesen K, Klunderud AT, Edvardsen RB et al (2011) The genome sequence of Atlantic cod reveals a unique immune system. Nature 477: 207-210 doi: 10.1038/nature10342
|
Suetake H, Araki K, Akatsu K, Somamoto T, Dijkstra JM, Yoshiura Y, Kikuchi K, Suzuki Y (2007) Genomic organization and expression of CD8α and CD8β genes in fugu Takifugu rubripes. Fish Shellfish Immunol 23: 1107-1118 doi: 10.1016/j.fsi.2007.05.005
|
Sugamata R, Suetake H, Kikuchi K, Suzuki Y (2009) Teleost B7 expressed on monocytes regulates T cell responses. J Immunol 182: 6799-6806 doi: 10.4049/jimmunol.0803371
|
Sun X, Shang N, Hu W, Wang Y, Guo Q (2007) Molecular cloning and characterization of carp (Cyprinus carpio L. ) CD8β and CD4-like genes. Fish Shellfish Immunol 23: 1242-1255 doi: 10.1016/j.fsi.2007.06.004
|
Tafalla C, Leal E, Yamaguchi T, Fischer U (2016) T cell immunity in the teleost digestive tract. Dev Comp Immunol 64: 167-177 doi: 10.1016/j.dci.2016.02.019
|
Takizawa F, Dijkstra JM, Kotterba P, Korytar T, Kock H, Kollner B, Jaureguiberry B, Nakanishi T, Fischer U (2011) The expression of CD8α discriminates distinct T cell subsets in teleost fish. Dev Comp Immunol 35: 752-763 doi: 10.1016/j.dci.2011.02.008
|
Takizawa F, Magadan S, Parra D, Xu Z, Korytár T, Boudinot P, Sunyer JO (2016) Novel teleost CD4-bearing cell populations provide insights into the evolutionary origins and primordial roles of CD4+ lymphocytes and CD4+ macrophages. J Immunol 196: 4522-4535 doi: 10.4049/jimmunol.1600222
|
Tang X, Qin Y, Sheng X, Xing J, Zhan W (2017) Characterization of CD3+ T lymphocytes of Japanese flounder (Paralichthys olivaceus) and its response after immunization with formalin-inactivated Edwardsiella tarda. Fish Shellfish Immunol 63: 220-227 doi: 10.1016/j.fsi.2017.02.024
|
Taylor EB, Wilson M, Bengten E (2015) The Src tyrosine kinase Lck binds to CD2, CD4-1, and CD4-2 T cell co-receptors in channel catfish, Ictalurus punctatus. Mol Immunol 66: 126-138 doi: 10.1016/j.molimm.2015.02.023
|
Terabe M, Tagaya Y, Zhu Q, Granger L, Roederer M, Waldmann TA, Berzofsky JA (2008) IL-15 expands unconventional CD8ααNK1.1+ T cells but not Vα14Jα18+ NKT cells1. J Immunol 180: 7276-7286 doi: 10.4049/jimmunol.180.11.7276
|
Tian H, Xing J, Tang X, Chi H, Sheng X, Zhan W (2021) Identification and characterization of a master transcription factor of Th1 cells, T-bet, within flounder (Paralichthys olivaceus). Front Immunol 12: 704324 doi: 10.3389/fimmu.2021.704324
|
Timmusk S, Jansson E, Pilstrom L (2003) The generation of monoclonal antibodies by genetic immunisation: antibodies against trout TCRα and IgL isotypes. Fish Shellfish Immunol 14: 187-206 doi: 10.1006/fsim.2002.0429
|
Toda H, Shibasaki Y, Koike T, Ohtani M, Takizawa F, Ototake M, Moritomo T, Nakanishi T (2009) Alloantigen-specific killing is mediated by CD8-positive T cells in fish. Dev Comp Immunol 33: 646-652 doi: 10.1016/j.dci.2008.11.008
|
Toda H, Saito Y, Koike T, Takizawa F, Araki K, Yabu T, Somamoto T, Suetake H, Suzuki Y, Ototake M, Moritomo T, Nakanishi T (2011) Conservation of characteristics and functions of CD4 positive lymphocytes in a teleost fish. Dev Comp Immunol 35: 650-660 doi: 10.1016/j.dci.2011.01.013
|
Tran HB, Risky PN, Padgett SRM, Lee YH, Chaung HC, Cheng TC (2020) Molecular characterization of cobia (Rachycentron canadum) CD4 homologues revealed the first evidence of soluble CD4 in fish. Fish Shellfish Immunol 99: 239-242 doi: 10.1016/j.fsi.2020.02.017
|
Triebel F, Jitsukawa S, Baixeras E, Roman-Roman S, Genevee C, Viegas-Pequignot E, Hercend T (1990) LAG-3, a novel lymphocyte activation gene closely related to CD4. J Exp Med 171: 1393-1405 doi: 10.1084/jem.171.5.1393
|
Turner JM, Brodsky MH, Irving BA, Levin SD, Perlmutter RM, Littman DR (1990) Interaction of the unique N-terminal region of tyrosine kinase p56lck with cytoplasmic domains of CD4 and CD8 is mediated by cysteine motifs. Cell 60: 755-765 doi: 10.1016/0092-8674(90)90090-2
|
Ulmer JB, Otten GR (2000) Priming of CTL responses by DNA vaccines: direct transfection of antigen presenting cells versus cross-priming. Dev Biol 104: 9-14
|
Veilette A, Bookman MA, Horak EM, Bolen JB (1988) The CD4 and CD8 T cell surface antigens are associated with the internal membrane tyrosine-protein kinase p56lck. Cell 55: 301-308 doi: 10.1016/0092-8674(88)90053-0
|
Wan YY, Flavell RA (2009) How diverse—CD4 effector T cells and their functions. J Mol Cell Biol 1: 20-36 doi: 10.1093/jmcb/mjp001
|
Wan F, Hu C, Ma J, Gao K, Xiang L, Shao J (2017) Characterization of γδ T cells from zebrafish provides insights into their important role in adaptive humoral immunity. Front Immunol 7: 675
|
Wang T, Holland JW, Martin S, Secombes CJ (2010) Sequence and expression analysis of two T helper master transcription factors, T-bet and GATA3, in rainbow trout Oncorhynchus mykiss and analysis of their expression during bacterial and parasitic infection. Fish Shellfish Immunol 29: 705-715 doi: 10.1016/j.fsi.2010.06.016
|
Wen Y, Fang W, Xiang LX, Pan RL, Shao JZ (2011) Identification of Treg-like cells in Tetraodon: insight into the origin of regulatory T subsets during early vertebrate evolution. Cell Mol Life Sci 68: 2615-2626 doi: 10.1007/s00018-010-0574-5
|
Wilkinson R, Barton M, Kotlarski I (1995) Identification of koala T lymphocytes using an anti-human CD3 antibody. Dev Comp Immunol 19: 537-545 doi: 10.1016/0145-305X(95)00023-M
|
Williams NS, Engelhard VH (1996) Identification of a population of CD4+ CTL that utilizes a perforin-rather than a Fas ligand dependent cytotoxic mechanism. J Immunol 156: 153-159
|
Williams AF, Davis SJ, He Q, Barclay AN (1989) Structural diversity in domains of the immunoglobulin superfamily. Cold Spring Harb Symp Quant Biol 54: 637-647 doi: 10.1101/SQB.1989.054.01.075
|
Xing J, Ma J, Tang X, Sheng X, Zhan W (2017a) Characterizations of CD4-1, CD4-2 and CD8β T cell subpopulations in peripheral blood leucocytes, spleen and head kidney of Japanese flounder (Paralichthys olivaceus). Mol Immunol 85: 155-165 doi: 10.1016/j.molimm.2017.02.015
|
Xing J, Xiao Y, Tang X, Sheng X, Zhan W (2017b) Inhibition of Cyclosporine A or rapamycin on T lymphocyte counts and the influence on the immune responses of B lymphocytes in flounder (Paralichthys olivaceus). Fish Shellfish Immunol 66: 78-85 doi: 10.1016/j.fsi.2017.05.017
|
Xing J, Tian H, Wang L, Tang X, Sheng X, Zhan W (2018a) Characterization of CD40+ leukocytes in flounder (Paralichthys olivaceus) and its response after hirame novirhabdovirus infection and immunization. Mol Immunol 104: 79-89 doi: 10.1016/j.molimm.2018.11.001
|
Xing J, Wang L, Tang X, Sheng X, Zhan W (2018b) Variations of T and B lymphocytes of flounder (Paralichthys olivaceus) after hirame novirhabdovirus infection and immunization. Mol Immunol 96: 19-27 doi: 10.1016/j.molimm.2018.02.007
|
Xing J, Luo K, Xiao Y, Tang X, Zhan W (2019a) Influence of CD4-1+, CD4-2+ and CD8+ T lymphocytes subpopulations on the immune response of B lymphocytes in flounder (Paralichthys olivaceus) immunized with thymus-dependent or thymus-independent antigen. Fish Shellfish Immunol 84: 979-986 doi: 10.1016/j.fsi.2018.11.004
|
Xing J, Xu H, Tang X, Sheng X, Zhan W (2019b) A DNA vaccine encoding the VAA gene of Vibrio anguillarum induces a protective immune response in flounder. Front Immunol 10: 499 doi: 10.3389/fimmu.2019.00499
|
Xing J, Tian H, Tang X, Sheng X, Zhan W (2020) Kinetics of T lymphocyte subsets and B lymphocytes in response to immunostimulants in flounder (Paralichthys olivaceus): implications for CD4+ T lymphocyte differentiation. Sci Rep 10: 13827 doi: 10.1038/s41598-020-69542-6
|
Xu J, Foy TM, Laman JD, Elliott EA, Dunn JJ, Waldschmidt TJ, Elsemore J, Noelle RJ, Flavell RA (1994) Mice deficient for the CD40 ligand. Immunity 1: 423-431 doi: 10.1016/1074-7613(94)90073-6
|
Yamasaki M, Araki K, Nakanishi T, Nakayasu C, Yamamoto A (2014) Role of CD4+ and CD8α+ T cells in protective immunity against Edwardsiella tarda infection of ginbuna crucian carp, Carassius auratus langsdorfii. Fish Shellfish Immunol 36: 299-304 doi: 10.1016/j.fsi.2013.11.016
|
Yoon S, Mitra S, Wyse C, Alnabulsi A, Zou J, Weerdenburg EM, Sar AM, Wang D, Secombes CJ, Bird S (2015) First demonstration of antigen induced cytokine expression by CD4-1+ lymphocytes in a poikilotherm: studies in zebrafish (Danio rerio). PLoS ONE 10: e0126378 doi: 10.1371/journal.pone.0126378
|
Zamoyska R (1994) The CD8 coreceptor revisited: one chain good, two chains better. Immunity 1: 243-246 doi: 10.1016/1074-7613(94)90075-2
|
Zhang YA, Hikima JI, Li J, Lapatra SE, Luo YP, Sunyer JO (2009) Conservation of structural and functional features in a primordial CD80/86 molecule from rainbow trout (Oncorhynchus mykiss), a primitive teleost fish. J Immunol 183: 83-96 doi: 10.4049/jimmunol.0900605
|
Zhang X, Zhang X, Wang P, Zhang Y (2018) Identification of another primordial CD80/86 molecule in rainbow trout: insights into the origin and evolution of CD80 and CD86 in vertebrates. Dev Comp Immunol 89: 73-82 doi: 10.1016/j.dci.2018.08.007
|
Zhou L, Chong M, Littman DR (2009) Plasticity of CD4+ T cell lineage differentiation. Immunity 30: 646-655 doi: 10.1016/j.immuni.2009.05.001
|
Zhu J, Paul WE (2010) CD4+ T cell plasticity—Th2 cells join the crowd. Immunity 32: 11-13 doi: 10.1016/j.immuni.2010.01.001
|
Zhu DM, Dustin ML, Cairo CW, Thatte HS, Golan DE (2006) Mechanisms of cellular avidity regulation in CD2-CD58-mediated T-cell adhesion. ACS Chem Biol 1: 649-658 doi: 10.1021/cb6002515
|
Zhu J, Yamane H, Paul WE (2010) Differentiation of effector CD4 T cell populations. Annu Rev Immunol 28: 445-489 doi: 10.1146/annurev-immunol-030409-101212
|
Zola H, Swart B, Boumsell L, Mason DY (2003) Human leucocyte differentiation antigen nomenclature: update on CD nomenclature. Report of IUIS/WHO subcommittee. J Immunol Methods 275: 1-8 doi: 10.1016/S0022-1759(03)00057-7
|
Zola H, Swart B, Nicholson I, Aasted B, Bensussan A, Boumsell L, Buckley C, Clark G, Drbal K, Engel P (2005) CD molecules 2005: human cell differentiation molecules. Blood 106: 3123-3126
|
Marker molecules | Fish species | Type of antibody | Application | References |
CD3ɛ | Salmon | pAb | WB, FCM, IHC, IFA | Koppang et al. (2010) |
Trout | mAb | Co-IP, FCM, IFA | Boardman et al. (2012) | |
Salmon | pAb | WB, FCM, IFA | Maisey et al. (2016) | |
Flounder | pAb | Co-IP, FCM, IFA, IHC | Tang et al. (2017) | |
Flounder | mAb | WB, FCM, IFA, FACS | Jung et al. (2017) | |
CD4-1 | Spotted green pufferfish | pAb | WB | Wen et al. (2011) |
Ginbuna crucian carp | mAb | WB, FCM, IFA, FACS | Toda et al. (2011) | |
Japanese pufferfish | pAb | WB, FCM, IFA, FACS | Kono and Korenaga (2013) | |
Zebrafish | pAb | WB, IFA, IHC, FCM, FACS | Yoon et al. (2015) | |
Trout | mAb | WB, FCM, IFA, FACS | Takizawa et al. (2016) | |
Trout | pAb | WB, FCM, IFA, FACS | Maisey et al. (2016) | |
Flounder | pAb mAb |
WB, FCM, IFA, IHC, FACS WB, FCM, IFA, FACS |
Xing et al.(2017a, b) Xing et al. (2020) |
|
Ginbuna crucian carp | pAb | IFA | Kato et al. (2019) | |
Flounder | mAb | WB, FCM, IFA, FACS | Jung et al. (2020a) | |
CD4-2 | Spotted green pufferfish | pAb | WB, FCM, IHC, FACS | Wen et al. (2011) |
Trout | mAb | WB, FCM, IFA, FACS | Takizawa et al. (2016) | |
Flounder | pAb mAb |
WB, FCM, IFA, IHC, FACS WB, FCM, IFA, FACS |
Xing et al.(2017a, b) Xing et al. (2020) |
|
Flounder | mAb | WB, FCM, IFA, FACS | Jung et al. (2020b) | |
CD8α | Torafugu | pAb | WB, Co-IP, FCM, FACS | Araki et al. (2008) |
Ginbuna crucian carp | mAb | WB, FCM, IFA, FACS | Toda et al. (2009) | |
Salmon | mAb | IHC | Hetland et al. (2010) | |
Orange-spotted grouper | pAb | WB, FCM, IFA | Chang et al. (2011) | |
Trout | mAb | WB, Co-IP, FCM, IFA, IHC, FACS | Takizawa et al. (2011) | |
Flounder | mAb | WB, FCM, IFA, FACS | Jung et al. (2021) | |
CD8β | Flounder | pAb | WB, FCM, IFA, IHC, FACS | Xing et al.(2017a, b) |
Flounder | mAb | WB, FCM, IFA, FACS | Jung et al. (2021) | |
Co-IP Co-immunoprecipitation, WB Western blotting, FCM Flow cytometry, IFA Immunofluorescence assay, IHC Immunohistochemistry, FACS Fluorescence activated cell sorting |