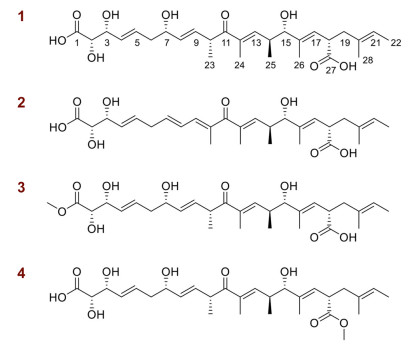
Citation: | Guihong Yu, Peng Sun, Reyilamu Aierken, Chunxiao Sun, Zhenzhen Zhang, Qian Che, Guojian Zhang, Tianjiao Zhu, Qianqun Gu, Mingyu Li, Dehai Li. 2022: Linear polyketides produced by co-culture of Penicillium crustosum and Penicillium fellutanum. Marine Life Science & Technology, 4(2): 222-236. DOI: 10.1007/s42995-021-00125-8 |
Linear polyketides are a large family with various bioactivities, such as antibacterial activity (Zander et al. 2011), antifungal activity (Bloor 2008) and anti-tumor activity (Yamaguchi et al. 2014). However, the determination of their absolute configurations is a challenge and has hindered their further application, mainly due to the flexibility of the carbon chain, the crowding of chiral centrals and/or the instability of structure. Until now, the absolute configuration of many linear polyketides still have not been determined, including actinopolysporins (Zhao et al. 2011), woodylides (Yu et al. 2012), phomenoic acid (Devys et al. 1984), arthrinic acid (Bloor 2008) and their derivatives (Zhao et al. 2019). Thus, it is urgent to explore a convenient strategy for determining the absolute configuration of linear polyketides.
During our search for novel bioactive secondary metabolites from deep-sea-derived microorganisms (Yu et al. 2019; Zhang et al. 2016), the co-culture strategy was used to active silent genes, among which the co-culture of Antarctic deepsea-derived fungus Penicillium crustosum PRB-2 and the Indian Ocean deep-sea-derived fungus Penicillium fellutanum HDN14-323 were found to produce two new peaks in the UV-profile, which led to the isolation of two new linear polyketides, penifellutins A (1) and B (2). Meanwhile, two esterification products of 1, penifellutins C (3) and D (4) were obtained because compound 1 could be esterified spontaneously when stored in methanol. Their configurations were difficult to determine because of the co-existence of three negative factors: chiral central crowdedness, structural flexibility and instability. Taking compound 1 as an example, it has seven chiral centers and five double bonds; the overabundance of hydroxyl and carboxyl groups increases the difficulty of configurational determination. For instance, the configurations of 1, 2-diols can be easily determined by making acetonide derivatives and performing Mo2(OAc)4-based CD experiments (Liu et al. 2009), but the adjacent carboxylic acid group and hydroxyl group in compound 1 become the negative factors. To avoid those factors, we esterified 1 before making an acetonide derivative and transformed compound 1 to compound 2, which loses OH-7, to perform the Mo2(OAc)4-based CD experiment. We also comprehensively used Mosher's method (Seco et al. 2004), the PGME method (Yabuuchi and Kusumi 2000), density functional theory calculation of 13C NMR and DP4 + probability analysis to determine the other configurations of compound 1 (Grimblat et al. 2015; Li et al. 2020), including the multiple hydroxyls, carboxyls, and methyls. As this kind of circumstance in compound 1 commonly occurs in other linear polyketides, such as the actinopolysporins, woodylides, phomenoic acid, arthrinic acid and their derivatives, our work, therefore, supplies a useful strategy for configurational determination. In addition, the anti-tumor activity of compounds 1–4 was evaluated in the doxycycline hyclate-induced zebrafish tumor model, where 1 and 2 showed inhibitory activity on the liver hyperplasia of zebrafish larvae at a concentration of 10 μmol/L. Here, we report the detailed isolation, structure elucidation, absolute configuration determination strategy and bioactivity evaluation of compounds 1–4 in the present work.
P. crustosum PRB-2 and P. fellutanum HDN14-323 were co-cultured in 15 cm Petri dishes containing 45 ml of fungal solid medium (Yu et al. 2019) for three weeks at 28 ℃. Compared to the mono-culture, two new peaks were appeared in the LC-UV profile (Supplementary Fig. S1). To isolate those two compounds, the EtOAc extract (6 g) from the co-cultured mycelia and the solid medium (3 L) was subjected to ODS MPLC to obtain nine subfractions; further purification using semi-preparative HPLC led to the isolation of compounds 1–2 (Fig. 1). As compound 1 could be esterified spontaneously when stored in methanol, two esterification products of 1, namely 3 and 4 (Fig. 1), were purified using semi-preparative HPLC.
Compounds 1 and 2 were both isolated as pale yellow oils, with molecular formulae of C28H42O9 and C28H40O8 deduced from the HREIMS peaks at m/z 521.2755 [M–H]- and m/z 503.2656 [M–H]-, respectively. Compound 1 can spontaneously transform into compound 2, suggesting that they have similar structures (Supplementary Fig. S2). The 1D NMR spectroscopic data indicate that 1 possesses six sp2 nonprotonated carbons, seven sp2 methines, seven sp3 methines, two sp3 methylenes and six methyls. By contrast, compound 2 lacks two sp3 methines including one oxygenated, while possessing one more sp2 nonprotonated carbon and one more sp2 methine, suggesting compound 2 has lost one molecule of water compared to compound 1 (Table 1). The COSY correlations of 1 between H-2 and H-3, H-10 and H3-23, H-14 and H-13/H-15/H3-25, H-18 and H-17/H2-19, and H-21 and H3-22 revealed many partial structures (Supplementary Fig. S3), while its HMBC correlations from H-2 to C-1, H-3 to C-4/C-5, H-7 to C-5/C-6/C-8/C-9, H-8 to C-10, H3-23 to C-9/C-11, H3-24 to C-11/C-12/C-13, H3-25 to C-13/C-15, H3-26 to C-15/C-16/C-17, H-18 to C-16/C- 20/C-27, H3-28 to C-19/C-20/C-21 and H3-22 to C-20 confirmed a polysubstituted linear structure. Furthermore, the chemical shifts of C-1 (δC 174.3), C-2 (δC 74.7), C-3 (δC 73.3), C-7 (δC 70.9), C-15 (δC 80.2) and C-27 (δC 175.3) in 1 along with the molecular weight indicated the existence of four hydroxyl and two carboxylic acid groups, which led to the final determination of its planar structure. Similarly, the planar structure of 2 was also determined by 2D NMR spectra and molecular weight. The only difference between 2 and 1 was the loss of one molecule of water at C-7/C- 10 in 2 to generate two new double bonds at C-7/C-8 and C-9/C-10 (Supplementary Fig.S3). In addition, the double bonds in compounds 1 and 2 can be determined as all E configurations by the NOESY correlations (Supplementary Fig. S3).
No | 1 | 2 | 3 | 4 | |||||||
δC | δH (J in Hz) | δC | δH (J in Hz) | δC | δH (J in Hz) | δC | δH (J in Hz) | ||||
1 | 174.3, C | 174.3, C | 173.1, C | 174.3, C | |||||||
2 | 74.7, CH | 3.81 d (3.4) | 74.6, CH | 3.87 d (3.4) | 75.2, CH | 3.90 dd (4.1, 6.7) | 74.7, CH | 3.82 d (3.1) | |||
3 | 73.3, CH | 4.13 dd (6.0, 3.3) | 73.1, CH | 4.22 t (5.0) | 73.5, CH | 4.09 m | 73.3, CH | 4.14 dd (5.0, 3.9) | |||
4 | 132.7, CH | 5.53 overlap | 132.6, CH | 5.58 dd (15.8, 6.1) | 132.2, CH | 5.46 overlap | 132.8, CH | 5.55 overlap | |||
5 | 128.1, CH | 5.56 overlap | 128.1, CH | 5.66 dt (15.5, 6.3) | 128.5, CH | 5.54 overlap | 128.1, CH | 5.56 overlap | |||
6 | 41.0, CH2 | 2.06 overlap | 35.7, CH2 | 2.92 t (6.4) | 41.0, CH2 | 2.05 overlap | 41.1, CH2 | 2.07 overlap | |||
7 | 70.9, CH | 3.87 m | 140.6, CH | 6.08 dt (15.1, 6.6) | 70.9, CH | 3.85 m | 70.9, CH | 3.87, m | |||
8 | 134.8, CH | 5.48 overlap | 127.2, CH | 6.47 dd (11.3, 15.2) | 134.8, CH | 5.46 overlap | 134.9, CH | 5.47 dd (5.8, 15.6) | |||
9 | 130.3, CH | 5.53 overlap | 139.9, CH | 6.80 d (11.1) | 130.3, CH | 5.51 overlap | 130.3, CH | 5.52 overlap | |||
10 | 42.0, CH | 3.96 m | 133.9, C | 42.0, CH | 3.96 m | 42.0, CH | 3.95 m | ||||
11 | 202.8, C | 200.7, C | 202.8, C | 202.7, C | |||||||
12 | 134.8, C | 134.4, C | 134.8, C | 134.8, C | |||||||
13 | 147.4, CH | 6.67 d (9.1) | 145.2, CH | 5.92 d (9.5) | 147.4, CH | 6.67 d (9.0) | 147.0, CH | 6.67 d (9.5) | |||
14 | 37.7, CH | 2.61 m | 37.2, CH | 2.60 m | 37.7, CH | 2.60 m | 37.6, CH | 2.64 m | |||
15 | 80.2, CH | 3.73 d (7.4) | 80.7, CH | 3.65 d (6.0) | 80.2, CH | 3.73 dd (4.0, 7.4) | 79.7, CH | 3.76 d (6.7) | |||
16 | 139.0, C | 139.2, C | 139.0, C | 139.8, C | |||||||
17 | 125.1, CH | 5.21 d (9.6) | 125.2, CH | 5.20 d (10.0) | 125.1, CH | 5.20 overlap | 123.9, CH | 5.24 d (9.5) | |||
18 | 43.2, CH | 3.28 m | 43.2, CH | 3.32 m | 43.2, CH | 3.28 m | 43.0, CH | 3.40 m | |||
19 | 43.0, CH2 | 2.04 overlap | 42.9, CH2 | 2.04 dd (7.9, 13.6) | 43.0, CH2 | 2.03 overlap | 42.9, CH2 | 2.07, overlap | |||
2.31 dd (7.1, 13.6) | 2.32 dd (6.7, 13.6) | 2.31 dd (7.1, 13.6) | 2.32 dd (7.4, 13.5) | ||||||||
20 | 133.0, C | 133.0, C | 133.0, C | 132.7, C | |||||||
21 | 120.7, CH | 5.16 q (7.2) | 120.7, CH | 5.16 m | 120.7, CH | 5.16 overlap | 121.0, CH | 5.16 q (6.6) | |||
22 | 13.7, CH3 | 1.46 d (6.7) | 13.7, CH3 | 1.47 d (6.6) | 13.7, CH3 | 1.46 d (6.6) | 13.7, CH3 | 1.47 d (6.6) | |||
23 | 18.3, CH3 | 1.03 d (6.7) | 13.2, CH3 | 1.83 s | 18.3, CH3 | 1.03 d (6.7) | 18.3, CH3 | 1.04 d (6.7) | |||
24 | 12.1, CH3 | 1.65 s | 13.6, CH3 | 1.73 s | 12.1, CH3 | 1.65 s | 12.0, CH3 | 1.65 s | |||
25 | 16.9, CH3 | 0.79 d (6.8) | 17.0, CH3 | 0.77 d (6.7) | 16.9, CH3 | 0.79 d (6.8) | 17.1, CH3 | 0.84 d (6.7) | |||
26 | 12.0, CH3 | 1.56 s | 11.9, CH3 | 1.60 s | 12.0, CH3 | 1.56 s | 12.4, CH3 | 1.57 s | |||
27 | 175.3, C | 175.3, C | 175.3, C | 174.2, C | |||||||
28 | 15.8, CH3 | 1.52 s | 15.8, CH3 | 1.54 s | 15.8, CH3 | 1.53 s | 15.8, CH3 | 1.53 s | |||
1-OCH3 | 51.7, CH3 | 3.59 s | |||||||||
27-OCH3 | 51.8, CH3 | 3.51 s | |||||||||
2-OH | – | – | 5.19 overlap | – | |||||||
3-OH | – | – | 4.86 overlap | – | |||||||
7-OH | – | – | 4.68 d (4.8) | – | |||||||
15-OH | – | – | 4.87 overlap | – |
The stereoscopic configurations of compounds 1 and 2 were determined by a combination of multiple methods including chemical derivatization, Mo2(OAc)4-based CD experiments, density functional theory calculation of 13C NMR and DP4 + probability analysis. First, in order to determine the relative configurations of 2-OH and 3-OH, compound 1 was esterified to generate the methyl ester (1a) and then transformed to its acetonide derivative (1b) (Supplementary Fig. S4) (Liu et al. 2009). The NOE correlations between H-2 (δH 4.19, d 7.6) and H3-28 (δH 1.36, s) and between H-3 (δH 4.45, t 7.4) and H3-29 (δH 1.34, s) of compound 1b indicated the threo configuration of 2, 3- diol (Supplementary Fig. S5). Second, to determine the absolute configuration of 2-OH and 3-OH, 1a (0.5 mg) and Mo2(OAc)4 (0.6 mg) were mixed in DMSO and subjected to CD experiment (Liu et al. 2009), but there was no obvious Cotton effect induced, possibly because the OH-7 affected the complexation of Mo2(OAc)4 with the 2, 3-diol. As there is no OH-7 in compound 2, we tried to determine the absolute configuration of 2-OH and 3-OH using compound 2. Similarly, the esterification product (2a) (Supplementary Fig. S4) was obtained and subjected to CD experiment after mixing with Mo2(OAc)4. From the negative sign of the Cotton effect at 310 nm (Supplementary Fig. S6), the sign of the HO–C–C–OH torsional angle is known (Supplementary Fig. S7); thus, the absolute configurations of the chiral centers bearing hydroxyl groups, i.e., C-2 and C-3 were determined as 2S and 3R (Liu et al. 2009). When determining the absolute configurations of 7-OH and 15-OH, we used the esterified acetonide derivative (1b) from the previous step to react with R/S-MTPA-Cl to avoid the effect of the carboxylic acid group and 2, 3-OH and then obtained 1c (S-MTPA ester of 1 on C-7 and C-15) and 1d (R-MTPA ester of 1 on C-7 and C-15), respectively. The proton signals around C-7 and C-15 of both derivatives were assigned by 1D and 2D NMR data (Supplementary Table S1, Fig. S8) (Seco et al. 2004). The ΔδSR values of 1c and 1d were negative for H-2 ~ H-7, H-13, H-14, H3-24, H3-25 and positive for H-8, H-9, H-15, H17 ~ H2-19, H3-26, H3-28 (Supplementary Fig. S9), indicating the 7S, 15S configurations. Simultaneously, the absolute configuration of CH3-25, which is adjacent to 15-OH, can be determined by coupling constant and conformational analysis using Mosher ester 1c (Raju et al. 2012). A large coupling of J=8.9 Hz between H-14 and H-15 suggested an anti-relationship between these protons. These data together with NOESY correlations between H3-10ʹʹ and H3-24, H-13 and H-15, H3-25 and H-15/H-17, H-14 and H-17/H3-26 revealed an erythro configuration between Me-25 and the hydroxyl group at C-15 (Supplementary Figs. S8, S10), which suggested the S configuration at C-14. Similarly, the absolute configuration of C-18 can be determined by applying the phenyl glycine methyl ester (PGME) method (Yabuuchi and Kusumi 2000), with both derivatives assigned by 1D and 2D NMR data (Supplementary Table S2, Fig. S11). The ΔδH values (ΔδH = δS - δR) obtained from the S- and R-PGME amides (1e and 1f) assigned the S configuration of C-18 (Supplementary Fig. S12). Finally, the configuration of internal methyl CH3-25 was determined by 13C NMR calculations using the fragments 1g and 1h as the truncated model structures of 1. The experimental and calculated 13C NMR data were compared by DP4 + probability analysis (Supplementary Table S3, Fig. S13) and 1h with the high probability of 99.35% was predicted to be the correct one, which suggested the R configuration at C-10 (Grimblat et al. 2015; Li et al. 2020). Thus, the full absolute configurations of compound 1 were determined. As compound 1 could spontaneously transform into compound 2, the absolute configurations of compound 2 were considered to be the same as those of compound 1.
With the exception of compound 2, we found that compound 1 could also spontaneously transformed to another two compounds (Supplementary Fig. S2). Further purification led to the yield of compounds 3 and 4 as pale yellow oils with the same molecular formula (C29H44O9), established based on the HRESIMS ions detected at m/z 535.2919 [M–H]- and m/z 535.2910 [M–H]-, respectively. Further analysis of the 1D and 2D NMR spectra indicated that the structural difference between 3 (or 4) and 1 was the existence of signal for an additional methyl at COOH-1 (δH 3.59) (or at COOH-27 (δH 3.51)) (Table 1). The configurations of 3 and 4 were speculated to be the same with those of compound 1, which were also consistent with the NOESY correlations (Supplementary Fig. S3).
To determine which strain synthesized compounds 1 and 2, we analyzed the UPLC-MS data. The data showed that compounds 1 and 2 could be slightly detected by MS when P. fellutanum HDN14-323 was cultured alone, but the production was too low to observe in the LC-UV profile (Supplementary Fig. S14). In other words, the co-culture condition up-regulated the biosynthetic pathway of compounds 1 and 2 in P. fellutanum HDN14-323, which helped us find them in the LC-UV profile and led to further purification (Supplementary Fig. S1).
Zebrafish are an ideal model which have been widely used to carry out chemical screens because of their transparent embryo, rapid growth and development, high gene homology and similar organ development with humans (Yan et al. 2017). Doxycycline hyclate (Dox)-induced zebrafish tumor models have developed to be a mature method to evaluate anti-hepatoma activity (Huo et al. 2019; Li et al. 2019), so we used this method to test the activities of penifellutins A–D (1–4) in this study. By comparing the size and fluorescence intensity of liver tumors, we found that compounds 1 and 2 can inhibit liver hyperplasia of zebrafish larvae at a concentration of 10 μmol/L (Figs. 2A, B, 3), which suggests that they are promising lead compounds for a new antihepatoma drug (Gan et al. 2020). However, the esterification products of 1 (i.e., 3 and 4) showed no activity (Figs. 2C, D, 3), indicating that the two carboxyls in this kind of structure are very important active sites.
In summary, during our research on the chemical potential of deep-sea-derived fungi, two new linear polyketides, namely penifellutins A (1) and B (2), were isolated from a co-culture of the deep-sea-derived fungi P. crustosum PRB-2 and P. fellutanum HDN14-323. UPLC-MS data suggested that the co-culture condition up-regulated the biosynthetic pathway of compounds 1 and 2 in P. fellutanum HDN14-323, which helped us find and purify them. In addition, two esterification products of 1, penifellutins C (3) and D (4), were obtained because compound 1 could be esterified spontaneously when stored in methanol. The absolute configurations of these compounds were determined by comprehensively using of Mo2(OAc)4-based CD experiment, density functional theory calculation of 13C NMR, DP4 + probability analysis and a series of reactions, such as making acetonide derivative, Mosher's method and PGME method, which provide a useful strategy for the configuration determination of linear polyketides. The evaluation of their anti-hepatoma activity shows that compounds 1 and 2 have noteworthy inhibitory activities on the liver hyperplasia of zebrafish larvae at a concentration of 10 μmol/L, indicating the potential of developing them to be anti-hepatoma drugs. In addition, preliminary structure–activity analysis indicated that two carboxyls in the structure are very important active sites.
UPLC-MS was performed on Waters ACQUITY UPLC H-Class module coupled to a SQ Detector 2 mass spectrometer (Waters Corporation, Milford, MA, USA). Semipreparative HPLC was conducted using an ODS column (YMC-Pack ODS-A, 10 × 250 mm, 5 µm, 3 ml/min) (YMC Co., Ltd., Kyoto, Japan). MPLC was applied on a BonaAgela CHEETAHTM HP100 (Beijing Agela Technologies Co., Ltd. Beijing, China). UV spectra were measured by Waters 2487 (Waters Corporation, Milford, MA, USA). Optical rotations were measured by a JASCO P-1020 digital polarimeter (JASCO Corporation, Tokyo, Japan). HRESIMS and ESIMS were measured on a Thermo Scientific LTQ Orbitrap XL mass spectrometer (Thermo Fisher Scientific, Waltham, MA, USA) or Micromass Q-TOF ULTIMA GLOBAL GAA076 LC Mass spectrometer (Waters Corporation, Milford, MA, USA). NMR spectra were recorded on an Agilent 500 MHz DD2 spectrometer with TMS as an internal standard (Agilent Technologies Inc., Santa Clara, CA, USA). ECD spectra were measured on a JASCO J-815 spectropolarimeter (JASCO Corporation, Tokyo, Japan).
The isolation and identification of P. crustosum PRB-2 and P. fellutanum HDN14-323 have been previously described (Wu et al. 2012; Zhang et al. 2016). Those two fungi were deposited at the Key Laboratory of Marine Drugs, the Ministry of Education of China, School of Medicine and Pharmacy, Ocean University of China, Qingdao, China.
P. crustosum PRB-2 and P. fellutanum HDN14-323 were co-cultured for three weeks at 28 ℃ under static fermentation in 15 cm petri dishes containing 45 ml solid medium, the ingredients of which have been previously described (Yu et al. 2019). After incubation, the solid medium (total 3 L) with mycelium was extracted three times with 5 L EtOAcand concentrated under reduced pressure to give the crude extract (6.0 g).
The crude extract (6.0 g) was fractionated by MPLC with a gradient elution of MeOH-H2O (5–100%) and yielded nine subfractions (fractions 1–9). Guided by the UPLCMS analysis result of those subfractions, fractions 8 and 9 were selected and separated by semi-preparative HPLC eluted with MeOH-H2O (with 2/1000 trifluoroacetic acid) (65: 35), among which compound 1 (60.0 mg, tR 18.5 min) was purified from fraction 8, while compound 2 (15.0 mg, tR 26.5 min) was purified from fraction 9. In addition, compound 1 can transform to other compounds in MeOH (Supplementary Fig. S2), and we purified 3 (4.0 mg, tR 21.9 min) and 4 (5.0 mg, tR 22.5 min) from the conversion products by semi-preparative HPLC eluted with CH3CN–H2O (with 2/1000 trifluoroacetic acid) (0–2 min: 20:80, 2–30 min: from 20:80 to 70:30).
Pale yellow oil; [α]20D + 5.6 (c 0.1, MeOH); UV (MeOH) λmax (log ε): 202 (3.90), 236 (3.56) nm; 1H and 13C NMR data, see Table 1; HRESIMS m/z 521.2755 [M–H]- (calcd. for C28H41O9, 521.2756).
Pale yellow oil; [α]20D + 4.7 (c 0.1, MeOH); UV (MeOH) λmax (log ε): 237 (3.23), 285 (3.86) nm; 1H and 13C NMR data, see Table 1; HRESIMS m/z 503.2656 [M–H]- (calcd. for C28H39O8, 503.2650).
Pale yellow oil; [α]20D + 7.0 (c 0.1, MeOH); UV (MeOH) λmax (log ε): 203 (3.89), 235 (3.49) nm; 1H and 13C NMR data, see Table 1; HRESIMS m/z 535.2919 [M–H]- (calcd. for C29H43O9, 535.2913).
Pale yellow oil; [α]20D + 9.2 (c 0.1, MeOH); UV (MeOH) λmax (log ε): 204 (3.93), 237 (3.52) nm; 1H and 13C NMR data, see Table 1; HRESIMS m/z 535.2910 [M–H]- (calcd. for C29H43O9, 535.2913).
The Zebrafish embryos Tg (fabp10:rtTA; TRE: EGFPkrasV12) were incubated with 25 μmol/ml doxycycline hyclate (Dox, Sigma-Aldrich) from 48 hpf (hours post-fertilization, hpf) to 96 hpf. Then the larvae, which were normally developed and had similar fluorescence intensity, and liver tumor area were selected and separated into 12-well plates (20 per well) with 2 ml zebrafish embryo culture medium. Dox and the test compound solutions were added to each well with the finally concentrations of 25 μmol/ml and 10 μmol/L, respectively, and then further incubated at 28.5 ℃ for three days while avoiding light. At 7 dpf, the larvae were washed several times and anesthetized with 0.08% tricaine and imaged under stereo fluorescence microscope (M205-FCA, Leica). The size and fluorescence intensity of liver tumors were measured by software Image J and analyzed by software GraphPad Prism 5. Statistical analyses were carried out by one-way ANOVA and t-tests (Chew et al. 2014; Tsuji et al. 2014). All procedures have been approved by the Xiamen University Institutional Animal Care and Use Committee (Protocol XMULAC20160089, 10 March 2016).
To the solution of compounds 1 or 2 (5 mg) in MeOH (1 ml), excess TMS-CHN2 in n-hexane (300 µl) was added, and the mixtures were stirred at room temperature for 2 h. Then the reaction mixture was concentrated in vacuo and purified by semi-preparative HPLC (MeCN: H2O = 30–100%) to yield methyl ester 1a (the reaction was performed for five times, total obtained 7.5 mg) or 2a (1.5 mg). 1a: pale yellow oil, ESIMS m/z 549.3 [M–H]-. 2a: pale yellow oil, ESIMS m/z 531.3 [M–H]-.
Compound 1a (6 mg) was treated with pyridinium p-toluene sulfonate (9 mg), 2, 2-dimethyoxypropane (800 μl) and MeOH (200 μl), then stirred at 35 ℃ for 16 h. The reaction mixture was concentrated in vacuo and purified by semi-preparative HPLC (MeCN: H2O = 30–100%) to yield the acetonide 1b (3.0 mg). 1b: pale yellow oil, ESIMS m/z 589.3 [M–H]-; 1H NMR (500 MHz, DMSO-d6) δ 6.66 (d, J = 9.5 Hz, 1H), 5.73 (dt, J = 15.4, 7.0 Hz, 1H), 5.59–5.42 (m, 3H), 5.23 (d, J = 9.6 Hz, 1H), 5.16 (q, J = 6.7 Hz, 1H), 4.91 (d, J = 4.6 Hz, 1H), 4.78 (d, J = 4.8 Hz, 1H), 4.45 (t, J = 7.4 Hz, 1H), 4.19 (d, J = 7.5 Hz, 1H), 3.93 (m, 2H), 3.76 (t, J = 5.5 Hz, 1H), 3.67 (s, 3H), 3.51 (s, 3H), 3.40 (q, J = 7.6 Hz, 1H), 2.64 (m, 1H), 2.32 (dd, J = 13.6, 7.5 Hz, 1H), 2.20–2.02 (m, 3H), 1.64 (s, 3H), 1.57 (s, 3H), 1.53 (s, 3H), 1.47 (d, J=6.7 Hz, 3H), 1.36 (s, 3H), 1.34 (s, 3H), 1.04 (d, J = 6.7 Hz, 3H), 0.84 (d, J = 6.8 Hz, 3H).
The mixture of compound 1a (or 2a) (0.5 mg) and Mo2(OAc)4 (0.6 mg) which dissolved in DMSO (1 ml) was subjected to CD experiment. The CD spectrum was recorded at 30 min after mixing. Additionally, the inherent CD of compound 1a (or 2a) was subtracted.
The sample of compound 1b (1.5 mg each) was treated with (R)- or (S)-MTPA-Cl (50 mg) in dry pyridine-d5 (0.5 ml). After stirring for 0.5 h at room temperature, the reaction mixtures were evaporated under reduced pressure and purified by HPLC individually (CH3CN: H2O = 30–100%, 3 ml/ min) to obtain the (S)-MTPA ester (1c) and (R)-MTPA ester (1d), respectively.
(S)-MTPA ester (1c): pale yellow oil; ESIMS m/z 1045.4 [M + Na]+; 1H and 13C NMR data, see Supplementary Table S1.
(R)-MTPA ester (1d): pale yellow oil; ESIMS m/z 1045.4 [M + Na]+; 1H and 13C NMR data, see Supplementary Table S1.
The sample of compound 1 (4 mg each) was treated with PyBOP (12 mg), HOBT (3.2 mg), N-methylmorpholine (12 µl) and (R)-PGME (4.5 mg) or (S)-PGME (4.5 mg) in dry CDCl3 (0.5 ml). After stirring for 3 h at room temperature, the reaction mixtures were evaporated in vacuo and the R-PGME amide (1e) and S-PGME amide (1f) were purified by semi-preparative HPLC (CH3CN: H2O=30–100%, 3 ml/ min), respectively.
R-PGME amide (1e): pale yellow oil; ESIMS m/z 817.4 [M + H]+; 1H and 13C NMR data, see Supplementary Table S2.
S-PGME amide (1f): pale yellow oil; ESIMS m/z 817.4 [M + H]+; 1H and 13C NMR data, see Supplementary Table S2.
Conformational search for 1g and 1h was performed by Spartan 14 software using MMFF (Merck molecular force field) (Spartan 14 2013). The conformers within 10 kcal/ mol were re-optimized at the B3LYP/6‐311 + G (d, p) level using the Gaussian 09 program (Frisch et al. 2009). The harmonic vibrational frequencies were calculated at the same level. The Boltzmann‐weighted population was calculated based on their relative thermal free energy (ΔG) obtained from the re-optimization result. The conformations within 10 kJ/mol were subjected to 13C NMR calculation using the Gauge‐Independent Atomic Orbital (GIAO) method at the B3LYP/6‐311 + G(d, p) level in DMSO with the polarizable continuum model (PCM). The scaled chemical shift (δs) of each conformer was calculated based on the equation proposed by Sarotti et al. (Grimblat et al. 2015). The final chemical shifts were obtained by averaging the data of all the conformers according to their Boltzmann distribution at 298.15 K. The DP4 + probability of 1g and 1h was calculated on the basis of the shielding tensor data by the Excel sheet provided by Sarotti et al. (Grimblat et al. 2015; Li et al. 2020).
The online version contains supplementary material available at https://doi.org/10.1007/s42995-021-00125-8.
This work was financially supported by the National Natural Science Foundation of China (41806167, 81670709), Project funded by China Postdoctoral Science Foundation (2017M622286), Qingdao Postdoctoral Applied Research Project Financially Supported by Qingdao Municipal Bureau of Human Resource and Social Security, Laboratory for Marine Drugs and Bioproducts of Qingdao National Laboratory for Marine Science and Technology (LMDBKF201805), and High-level Talents Research Fund of Qingdao Agricultural University (Grants 665/1120034). We thank Dr. Zhiyuan Gong (National Univeristy of Singapore) for the gift of Tg (Fabp10-rtTA: TRE-eGFPKRASV12) transgenic line.
GY and PS contributed equally. DL and ML conceived and designed the experiments; GY and PS performed the experiments; RA, CS, ZZ, QC, GZ, TZ, QG participated in the experimental process and result discussion. GY analyzed the data and wrote the paper.
The authors declare that they have no conflict of interest.
This article does not contain any studies with human participants. All procedures on animals have been approved by the Xiamen University Institutional Animal Care and Use Committee (Protocol XMULAC20160089, 10 March 2016).
Bloor S (2008) Arthrinic acid, a novel antifungal polyhydroxyacid from Arthrinium phaeospermum. J Antibiot 61: 515–517 doi: 10.1038/ja.2008.69
|
Chew TW, Liu XJ, Liu L, Spitsbergen JM, Gong Z, Low BC (2014) Crosstalk of Ras and Rho: activation of RhoA abates Kras-induced liver tumorigenesis in transgenic zebrafish models. Oncogene 33: 2717–2727 doi: 10.1038/onc.2013.240
|
Devys M, Ferezou JP, Topgi RS, Barbier M, Bousquet JF, Kollmann A (1984) Structure and biosynthesis of phomenoic acid, an antifungal compound isolated from Phoma lingam Tode. J Chem Soc, Perkin Trans 1: 2133–2137
|
Frisch MJ, Trucks GW, Schlegel HB, Scuseria GE, Robb MA, Cheeseman JR, Scalmani G, Barone V, Mennucci B, Petersson GA, Nakatsuji H, Caricato M, Li X, Hratchian HP, Izmaylov AF, Bloino J, Zheng G, Sonnenberg JL, Hada M, Ehara M et al (2009) Gaussian 09, Revision A. 1. Gaussian Inc., Wallingford, CT
|
Gan Q, Lin C, Lu C, Chang Y, Che Q, Zhang G, Zhu T, Gu Q, Wu Z, Li M, Li D (2020) Staprexanthones, xanthone-type stimulators of pancreatic β-cell proliferation from a mangrove endophytic fungus. J Nat Prod 83: 2996–3003 doi: 10.1021/acs.jnatprod.0c00535
|
Grimblat N, Zanardi MM, Sarotti AM (2015) Beyond DP4: an improved probability for the stereochemical assignment of isomeric compounds using quantum chemical calculations of NMR shifts. J Org Chem 80: 12526–12534 doi: 10.1021/acs.joc.5b02396
|
Huo X, Li H, Li Z, Yan C, Mathavan S, Liu J, Gong Z (2019) Transcriptomic analyses of oncogenic hepatocytes reveal common and different molecular pathways of hepatocarcinogenesis in different developmental stages and genders in kras(G12V) transgenic zebrafish. Biochem Biophys Res Commun 510: 558–564 doi: 10.1016/j.bbrc.2019.02.008
|
Li J, Chen M, Hao X, Li S, Li F, Yu L, Xiao C, Gan M (2020) Structural revision and absolute configuration of burnettramic acid A. Org Lett 22: 98–101 doi: 10.1021/acs.orglett.9b04008
|
Li Y, Agrawal I, Gong Z (2019) Reversion of tumor hepatocytes to normal hepatocytes during liver tumor regression in an oncogene-expressing transgenic zebrafish model. Dis Model Mech 12: dmm039578
|
Liu J, Liu Y, Si Y, Yu S, Qu J, Xu S, Hu Y, Ma S (2009) New vernocuminosides from the stem barks of Vernonia cumingiana Benth. Steroids 74: 51–61 doi: 10.1016/j.steroids.2008.09.005
|
Raju R, Gromyko O, Fedorenko V, Luzhetskyy A, Plaza A, Müller R (2012) Juniperolide A: a new polyketide isolated from a terrestrial actinomycete, Streptomyces sp. Org Lett 14: 5860–5863 doi: 10.1021/ol302766z
|
Seco JM, Quiñoá E, Riguera R (2004) The assignment of absolute configuration by NMR. Chem Rev 104: 17–118 doi: 10.1021/cr000665j
|
Spartan 14 W, Inc. : Irvine, CA, USA (2013)
|
Tsuji N, Ninov N, Delawary M, Osman S, Roh AS, Gut P, Stainier DYR (2014) Whole organism high content screening identifies stimulators of pancreatic beta-cell proliferation. PloS ONE 9: e104112 doi: 10.1371/journal.pone.0104112
|
Wu G, Ma H, Zhu T, Li J, Gu Q, Li D (2012) Penilactones A and B, two novel polyketides from Antarctic deep-sea derived fungus Penicillium crustosum PRB-2. Tetrahedron 68: 9745–9749 doi: 10.1016/j.tet.2012.09.038
|
Yabuuchi T, Kusumi T (2000) Phenylglycine methyl ester, a useful tool for absolute configuration determination of various chiral carboxylic acids. J Org Chem 65: 397–404 doi: 10.1021/jo991218a
|
Yamaguchi S, Uochi Y, Agatsuma T, Iwamoto S, Onodera H (2014) Manufacture of 4, 6-hexadecadiene-2, 4-dicarboxylic acid derivatives with Scopulariopsis. WO2014002967-A1
|
Yan C, Yang Q, Huo X, Li H, Zhou L, Gong Z (2017) Chemical inhibition reveals differential requirements of signaling pathways in kras(V12)- and Myc-induced liver tumors in transgenic zebrafish. Sci Rep 7: 45796 doi: 10.1038/srep45796
|
Yu H, Liu X, Xu Y, Gan J, Jiao W, Shen Y, Lin H (2012) Woodylides A–C, new cytotoxic linear polyketides from the south China sea sponge Plakortis simplex. Mar Drugs 10: 1027–1036 doi: 10.3390/md10051027
|
Yu G, Sun Z, Peng J, Zhu M, Che Q, Zhang G, Zhu T, Gu Q, Li D (2019) Secondary metabolites produced by combined culture of Penicillium crustosum and a Xylaria sp. J Nat Prod 82: 2013–2017 doi: 10.1021/acs.jnatprod.9b00345
|
Zander W, Gerth K, Mohr KI, Kessler W, Jansen R, Mueller R (2011) Roimatacene: an antibiotic against gram-negative bacteria isolated from Cystobacter ferrugineus Cb G35 (Myxobacteria). Chem Eur J 17: 7875–7881 doi: 10.1002/chem.201003677
|
Zhang Z, Guo W, He X, Che Q, Zhu T, Gu Q, Li D (2016) Peniphenylanes A-G from the deep-sea-derived fungus Penicillium fellutanum HDN14-323. Planta Med 82: 872–876 doi: 10.1055/s-0042-102885
|
Zhao L, Huang S, Tang S, Jiang C, Duan Y, Beutler JA, Henrich CJ, McMahon JB, Schmid T, Blees JS, Colburn NH, Rajski SR, Shen B (2011) Actinopolysporins A–C and tubercidin as a Pdcd4 stabilizer from the halophilic actinomycete Actinopolyspora erythraea YIM 90600. J Nat Prod 74: 1990–1995 doi: 10.1021/np200603g
|
Zhao Z, Ding W, Wang P-M, Zheng D, Xu J (2019) Five polyketides isolated from the marine-derived fungus Arthrinium sp. Nat Prod Res 35: 2470–2475
|
No | 1 | 2 | 3 | 4 | |||||||
δC | δH (J in Hz) | δC | δH (J in Hz) | δC | δH (J in Hz) | δC | δH (J in Hz) | ||||
1 | 174.3, C | 174.3, C | 173.1, C | 174.3, C | |||||||
2 | 74.7, CH | 3.81 d (3.4) | 74.6, CH | 3.87 d (3.4) | 75.2, CH | 3.90 dd (4.1, 6.7) | 74.7, CH | 3.82 d (3.1) | |||
3 | 73.3, CH | 4.13 dd (6.0, 3.3) | 73.1, CH | 4.22 t (5.0) | 73.5, CH | 4.09 m | 73.3, CH | 4.14 dd (5.0, 3.9) | |||
4 | 132.7, CH | 5.53 overlap | 132.6, CH | 5.58 dd (15.8, 6.1) | 132.2, CH | 5.46 overlap | 132.8, CH | 5.55 overlap | |||
5 | 128.1, CH | 5.56 overlap | 128.1, CH | 5.66 dt (15.5, 6.3) | 128.5, CH | 5.54 overlap | 128.1, CH | 5.56 overlap | |||
6 | 41.0, CH2 | 2.06 overlap | 35.7, CH2 | 2.92 t (6.4) | 41.0, CH2 | 2.05 overlap | 41.1, CH2 | 2.07 overlap | |||
7 | 70.9, CH | 3.87 m | 140.6, CH | 6.08 dt (15.1, 6.6) | 70.9, CH | 3.85 m | 70.9, CH | 3.87, m | |||
8 | 134.8, CH | 5.48 overlap | 127.2, CH | 6.47 dd (11.3, 15.2) | 134.8, CH | 5.46 overlap | 134.9, CH | 5.47 dd (5.8, 15.6) | |||
9 | 130.3, CH | 5.53 overlap | 139.9, CH | 6.80 d (11.1) | 130.3, CH | 5.51 overlap | 130.3, CH | 5.52 overlap | |||
10 | 42.0, CH | 3.96 m | 133.9, C | 42.0, CH | 3.96 m | 42.0, CH | 3.95 m | ||||
11 | 202.8, C | 200.7, C | 202.8, C | 202.7, C | |||||||
12 | 134.8, C | 134.4, C | 134.8, C | 134.8, C | |||||||
13 | 147.4, CH | 6.67 d (9.1) | 145.2, CH | 5.92 d (9.5) | 147.4, CH | 6.67 d (9.0) | 147.0, CH | 6.67 d (9.5) | |||
14 | 37.7, CH | 2.61 m | 37.2, CH | 2.60 m | 37.7, CH | 2.60 m | 37.6, CH | 2.64 m | |||
15 | 80.2, CH | 3.73 d (7.4) | 80.7, CH | 3.65 d (6.0) | 80.2, CH | 3.73 dd (4.0, 7.4) | 79.7, CH | 3.76 d (6.7) | |||
16 | 139.0, C | 139.2, C | 139.0, C | 139.8, C | |||||||
17 | 125.1, CH | 5.21 d (9.6) | 125.2, CH | 5.20 d (10.0) | 125.1, CH | 5.20 overlap | 123.9, CH | 5.24 d (9.5) | |||
18 | 43.2, CH | 3.28 m | 43.2, CH | 3.32 m | 43.2, CH | 3.28 m | 43.0, CH | 3.40 m | |||
19 | 43.0, CH2 | 2.04 overlap | 42.9, CH2 | 2.04 dd (7.9, 13.6) | 43.0, CH2 | 2.03 overlap | 42.9, CH2 | 2.07, overlap | |||
2.31 dd (7.1, 13.6) | 2.32 dd (6.7, 13.6) | 2.31 dd (7.1, 13.6) | 2.32 dd (7.4, 13.5) | ||||||||
20 | 133.0, C | 133.0, C | 133.0, C | 132.7, C | |||||||
21 | 120.7, CH | 5.16 q (7.2) | 120.7, CH | 5.16 m | 120.7, CH | 5.16 overlap | 121.0, CH | 5.16 q (6.6) | |||
22 | 13.7, CH3 | 1.46 d (6.7) | 13.7, CH3 | 1.47 d (6.6) | 13.7, CH3 | 1.46 d (6.6) | 13.7, CH3 | 1.47 d (6.6) | |||
23 | 18.3, CH3 | 1.03 d (6.7) | 13.2, CH3 | 1.83 s | 18.3, CH3 | 1.03 d (6.7) | 18.3, CH3 | 1.04 d (6.7) | |||
24 | 12.1, CH3 | 1.65 s | 13.6, CH3 | 1.73 s | 12.1, CH3 | 1.65 s | 12.0, CH3 | 1.65 s | |||
25 | 16.9, CH3 | 0.79 d (6.8) | 17.0, CH3 | 0.77 d (6.7) | 16.9, CH3 | 0.79 d (6.8) | 17.1, CH3 | 0.84 d (6.7) | |||
26 | 12.0, CH3 | 1.56 s | 11.9, CH3 | 1.60 s | 12.0, CH3 | 1.56 s | 12.4, CH3 | 1.57 s | |||
27 | 175.3, C | 175.3, C | 175.3, C | 174.2, C | |||||||
28 | 15.8, CH3 | 1.52 s | 15.8, CH3 | 1.54 s | 15.8, CH3 | 1.53 s | 15.8, CH3 | 1.53 s | |||
1-OCH3 | 51.7, CH3 | 3.59 s | |||||||||
27-OCH3 | 51.8, CH3 | 3.51 s | |||||||||
2-OH | – | – | 5.19 overlap | – | |||||||
3-OH | – | – | 4.86 overlap | – | |||||||
7-OH | – | – | 4.68 d (4.8) | – | |||||||
15-OH | – | – | 4.87 overlap | – |